Investigators' Blog

Cure Kids supporting research
At Cure Kids, we are dedicated to raising funds in order for research to be carried out that will best contribute to our vision of a healthy childhood for everyone. As the Research Manager, I am fortunate enough to have a close connection with all the research projects we fund. I’m also lucky to be able to connect with many of our wonderful ambassadors, many of whom live with the conditions that we’re researching.
In 2014, we became patently aware – via a proposal from Dr Siouxsie Wiles – about the imminent crisis that we (and by we, I mean, everyone) are facing. Over 80 years ago, health research was advanced immeasurably – and with it, health outcomes – when a beautiful collision of science and happenstance would result in the discovery of penicillin from the fungus, penicilium.
Dr Alexander Fleming, the scientist who discovered penicilium, had some ominous words, when accepting his Nobel Prize in 1945, of which we have not taken heed, ‘The thoughtless person playing with penicillin treatment is morally responsible for the death of the man who succumbs to infection with the penicillin-resistant organism’.
Dr Fleming, in equal parts wisdom and humility, knew the limitations of his momentous finding. The world has blindly careered into what may become a post-antibiotic era; to the point, now, where there are very few effective bulwarks against what were once treatable, innocuous bugs, but are now pernicious soldiers with an ever-increasing arsenal.
Antibiotics have been a great defence against unwanted visitors for decades. They have been used to prevent infections in routine surgeries, treat preterm babies, as well as provide a defence when patients undergo chemotherapy. The problem is that imprudent use is rendering antibiotics useless. We have all presumed this is a sustainable use of antibiotics; it is not.
Much like other collective-action efforts, such as action on climate change, antibiotic resistance has not been given the time of day – nor funding – it needs.
So many of our Cure Kids ambassadors have compromised immune systems, where they are vulnerable to the invasiveness of these drug-resistant bugs. Cystic fibrosis, a life-limiting inherited lung disorder is characterised by thick mucus build-up in the lungs. This mucus provides an amenable breeding ground for the bug Pseudomonas aeruginosa, with strains arising unperturbed by previous effective treatments.
A multi-pronged attack is required if we are to stave off countless deaths. While medical institutions attempt to be better stewards during prescription, Dr Wiles and her team are scouring the treasure trove of unique fungi located in New Zealand and the South Pacific. These untapped fungi could well hold a solution, and we’d like to do all we can to expedite the process of bringing the world back to an era where antibiotics are once again a powerful force against sophisticated superbugs.
Cure Kids occupy a space which deals with both the lab end, and the clinical end. We are excited to lend our support to Dr Wiles’ quest, as we are all too aware of the havoc these superbugs can wreak on our children. Us adults have reaped the benefits of advances enabled by Dr Fleming’s finding. It is now incumbent upon us to afford these same benefits to our young ones.
About
Ryan Chandler is Research Manager for Cure Kids – a charity funding vital medical research so everyone can live a healthy childhood. Donate to help.
What is InfectedNZ?
Hey, Aotearoa. It’s time we had a chat about infectious diseases and what we’re going to do about the looming antimicrobial armageddon. That’s why we’ve asked leading health, social and economic researchers, and people with personal stories, to help us get real about our vulnerability and discuss solutions. Follow their blogs right here at tepunhahamatatini.ac.nz and watch the conversation spread across social media with #infectedNZ.
Backing it all up, wherever possible, is data from the good folk at Figure.NZ. Their super duper charts are based on data sourced from public repositories, government departments, academics and corporations. Check out their #infectedNZ data board and sign-up to create your very own data board on any topic that floats your boat.

The costs of antimicrobial resistance
What might the economic costs of antibiotic/antimicrobial-resistant infections be in New Zealand? The simple answer is – we don’t know. As far as I’m aware, there’s been no New Zealand studies publicly disseminated on this topic. Therefore, we have to look overseas for reported studies.
While the US Centers for Disease Control and Prevention (2013) stated the total economic cost of antibiotic-resistant infections to the US economy was difficult to calculate, they cited year 2000 estimates provided by the Alliance for the Prudent Use of Antibiotics (APUA), an international non-governmental organisation. Based on ~900,000 cases of antibiotic-resistant infections a year, the APUA estimates ranged as high as US$20 billion in excess direct healthcare costs, with additional costs to society for lost productivity as high as US$35 billion a year. Given the number of cases reported has more than doubled over the past decade – CDC recently estimated that over 2,049,442 people are sickened every year with antibiotic-resistant infections, with at least 23,000 dying annually, who could once be easily cured – costs will have risen substantially. Furthermore, drug resistance related to viruses such as HIV and influenza was not included, nor was drug resistance among parasites such as those that cause malaria, so the true costs are likely even higher.
More recently, the UK government commissioned a review on antimicrobial resistance, in 2014, chaired by macroeconomist Jim O’Neill. As part of the two-year independent review, they estimated losses to the world economy caused by reductions to the size and efficiency of the labour force resulting from three resistant hospital-acquired infections (Escherichia coli, Klebsiella pneumoniae, and Staphylococcus aureus) and three major infectious diseases (HIV, tuberculosis, and malaria). By 2050, they projected that in a world without effective antimicrobial therapy, gross domestic product (GDP) per capita in high-income countries would be 2.3% lower each and every year compared to a world with no resistance. An older 2005 study led by the London School of Hygiene and Tropical Medicine (Richard Smith and colleagues) estimated current losses attributable to a level of MRSA (alone) of 40% in the UK at 0.4% to 1.6% of GDP – thus suggesting the review projections might be on the low side.
The review team recognised that their projected GDP losses will be an underestimation of the overall costs of antimicrobial resistance for several reasons. First, only six conditions were included as the research team was unable to identify sufficiently robust data on others. The worldwide extent of the problem is hard to pinpoint since data are incomplete, and there’s no standard for tracking such infections and associated deaths. That also makes totaling the economic impact — and exact death count — difficult to do. Second, people may choose not to undergo certain procedures because of the heightened risks involved, resulting in further morbidity and mortality. For instance, most intensive care medicine, and surgical procedures such as caesarean sections, organ transplantations, removing tumours, and hip replacements would not be possible if you don’t have effective antibiotics available that can deal with the infections that will result – modern medicine would be paralysed. And third, costs in the healthcare system and from reduced activity in travel and trade were also not included.
It’s possible we could slice and dice the costs above and apportion them to the New Zealand population. But the different methodological approaches taken, dissimilar healthcare systems, and varying usage of antibiotics in human and animal health may mean the results are not generalisable.
Given that the dangers of resistance are widely acknowledged, why hasn’t more been done in the past? One reason is that antibiotic resistance has fallen victim to evidence-based policy making, which prioritises health problems by economic burden and cost-effectiveness of interventions. While the estimated costs of antibiotic resistance are substantial, they have not been as high as in many (competing) therapeutic areas. Thus health economists have been unable to show that antibiotic resistance costs enough to be a health priority. However, the potential future costs of a world without effective antibiotics would be much larger than the cost of antimicrobial resistance today – one could draw an analogy with climate change – though it is currently not clear to what extent, or how quickly, the future burden of antimicrobial resistance will grow. The O’Neill review stated that antimicrobial-resistant infections (due to the six above conditions) are responsible for ~700,000 deaths globally each year, with this number likely to be an underestimate due to poor reporting and surveillance. By 2050, these deaths are projected to rise to 10 million a year: more than global annual cancer deaths.
International co-ordination towards the goals outlined in the Final Report and Recommendations of the Review on Antimicrobial Resistance (just published in May) is a way forward. In the words of an esteemed UK colleague, rather than see expenditure on antimicrobial policies as a cost, we should think of it as an insurance policy against a catastrophe; albeit one which we hope will never happen.
References
- US Centers for Disease Control and Prevention. Antibiotic resistance threats in the United States, 2013. Atlanta: CDC, Department of Health and Human Services. 2013.
- O’Neill J. Review on antimicrobial resistance. Antimicrobial Resistance: Tackling a Crisis for the Health and Wealth of Nations. 2014.
- O’Neill J. The review on antimicrobial resistance. Tackling Drug-Resistant Infections Globally: Final Report and Recommendations. 2016.
- Smith RD, Yago M, Millar M, Coast J. Assessing the macroeconomic impact of a healthcare problem: The application of computable general equilibrium analysis to antimicrobial resistance. Journal of Health Economics 2005; 24(6):1055–75.
About
William Leung is a lecturer in health economics at the University of Otago, Wellington, and is currently leading the economic evaluation of SHIVERS, a CDC-funded influenza project.
What is InfectedNZ?
Hey, Aotearoa. It’s time we had a chat about infectious diseases and what we’re going to do about the looming antimicrobial armageddon. That’s why we’ve asked leading health, social and economic researchers, and people with personal stories, to help us get real about our vulnerability and discuss solutions. Follow their blogs right here at tepunhahamatatini.ac.nz and watch the conversation spread across social media with #infectedNZ.
Backing it all up, wherever possible, is data from the good folk at Figure.NZ. Their super duper charts are based on data sourced from public repositories, government departments, academics and corporations. Check out their #infectedNZ data board and sign-up to create your very own data board on any topic that floats your boat.

On the hunt for bacteriophages
The crisis of antibiotic resistant superbugs has been creeping up on us for the past 88 years. Alexander Flemming, discoverer of penicillin, the first antibiotic, in that year, said it well when he received is Nobel prize:
“It is not difficult to make microbes resistant to penicillin in the laboratory by exposing them to concentrations not sufficient to kill them, and the same thing has occasionally happened in the body. The time may come when penicillin can be bought by anyone in the shops. Then there is the danger that the ignorant man may easily underdose himself and by exposing his microbes to non-lethal quantities of the drug make them resistant.”
The superbugs that threaten to drag us back into the pre-antibiotic era today were completely predictable from the very start.
One way to think about the antibiotics is to think of them as weapons in a war that is being waged between microbes all around us. Microbes have fought for time immemorial using antibiotics and they have evolved an equally impressive cache of countermeasures: genes that confer resistance to antibiotics.
When humans stumbled into this on-going battle, we had little appreciation for the collection of resistance mechanisms that already exist in nature. To make things worse, we severely underestimate the rates that these could be borrowed or repurposed across species boundaries very easily. This is a process we now know as horizontal gene transfer.
Given our current position, looking back at the on-going microbial melee for new potential allies in this struggle is warranted. In some parts of the world, including Russia and Georgia, the natural parasites of bacteria, Bacteriophages, have been put to use in medicine since before World War I.

Bacteriophages. At left, a diagram of a typical bacteriophage. At right, A bacteriophage underneath the electron microscope.
Bacteriophages (phages for short) were discovered roughly 100 years ago, before antibiotics and they have been used medicinally in the form of small liquid vials, full of mixed cocktails of bacteriophages. Bacteriophage therapy practitioners have designed these phage cocktails and used them to great effect while the rest of Western medicine pursued Flemming’s new antibiotics.
Bacteriophages are not currently accepted in Western medicine but they are approved for use in food preparation by the USDA. Bacteriophage therapy has many obstacles to overcome before it might be accepted. One of these is the double edged sword of specificity. Bacteriophages are highly specific to a particular bacterial strain. This means that they will not hurt the beneficial microbes that contribute to our health. However it also means that finding the right one for a particular infection requires a stockpile of phage types. We need to learn more about the bacteriophages around us before we will be ready for this sort of personalized approach to fighting infection. There are estimated to be 1031 bacteriophages on the planet so as long as we can find them and study them, we have access to the stockpiles we need.
In order to learn more about these important entities, the undergraduates that take our Bacteriophage Discover and Genomics paper at Massey University are contributing to the global pool of phage information. We have joined the Howard Hughes Medical Institute’s Science Education Alliance programme to hunt for phages that are able to infect a close cousin of Mycobacterium tuberculosis. Last year we found four phages and sequenced two. As far as we know these are the very first Mycobacteriophages to be found in New Zealand (below figure).

Phage Hunt Map. Each symbol represents a single sequenced Mycobacteriophage. Last year, the phage hunters at Massey University put NZ on the map!
To hear more about Bacteriophages, watch Heather’s TedX talk.
It’s an exciting time to be a phage hunter! To learn more about our phage hunt, check out the student blog, Phage Hunt NZ.
About
Dr Heather Hendrickson is a Senior Lecturer at Massey University. She has been dabbling in Phage Hunting since her time at the Pittsburgh Bacteriophage Institute as a PhD student. Heather’s laboratory at Massey University, Auckland studies the evolution of bacteria and bacteriophage discovery in Pseudomonas, Mycobacteria and Lactococcus.
What is InfectedNZ?
Hey, Aotearoa. It’s time we had a chat about infectious diseases and what we’re going to do about the looming antimicrobial armageddon. That’s why we’ve asked leading health, social and economic researchers, and people with personal stories, to help us get real about our vulnerability and discuss solutions. Follow their blogs right here at tepunhahamatatini.ac.nz and watch the conversation spread across social media with #infectedNZ.
Backing it all up, wherever possible, is data from the good folk at Figure.NZ. Their super duper charts are based on data sourced from public repositories, government departments, academics and corporations. Check out their #infectedNZ data board and sign-up to create your very own data board on any topic that floats your boat.

The 3Rs of antimicrobial resistance: going back to basics to avert a crisis
Down in Otago, researchers’ focus on microbiology fundamentals is leading New Zealand’s fight against drug resistant pathogens.
In May 2016 the UK government’s Review on Antimicrobial Resistance (AMR) published its final report, summarising the Review’s findings from two years of analysis. The conclusions, whilst welcomed by the scientific community, chimed with what leading researchers, such as the University of Otago’s Professor Greg Cook, have more or less been saying for over a decade. Namely, that AMR is already a global problem (including here in Aotearoa New Zealand) and that it will take a concerted effort with governments, scientists and pharmaceutical companies working together to solve it. In the meantime the report recommended taking steps that could help prevent the rise and spread of AMR. These, in essence, can be boiled down to the three R’s of antimicrobials: Refine, Reduce and Replace, and it is these three R’s that research in Prof Cook’s laboratory focuses on.
Refining the use of antibiotics basically means using the right drugs, to treat the right bugs, at the right time. In order to achieve that, healthcare workers need rapid diagnostic tests which will tell them whether the bug their patient has is already resistant to antibiotics, and if so, to which ones. The Cook lab is part of an international collaboration between Otago and South East Asia whereby the team is trying to establish whole genome sequencing (WGS) of the tuberculosis (TB)-causing bacterium, Mycobacterium tuberculosis, as a route to faster diagnoses. WGS allows researchers to analyse the genome of AMR bacteria, looking for genetic markers that indicate which antibiotics will work and those to which the bacterium is resistant.
The second of the three R’s, Reduce, is aimed at using fewer antibiotics to lower levels of AMR in our society and in the environment. An unfortunate Catch 22 experienced in antibiotic use is that, the more you use an antibiotic, the faster resistance will arise. Around the world there are many countries that use huge numbers of antibiotics in farming. In the Unites States alone roughly 70% of antimicrobials used each year are used on animals. Luckily for us, New Zealand is one of the lowest users of antibiotics in farming worldwide but still, there’s room for improvement.
In the dairy industry, when a cow’s udder becomes infected (know as mastitis) it’s more often than not due to a bacterial infection and treatment involves a course of antibiotics, such as penicillin. Moreover, whenever a cow is treated with antibiotics its milk can’t be used and needs to be discarded. Treatment and discarded milk cost the New Zealand dairy industry over $280 million a year. In this scenario, prevention really is better than the cure, for all of us. By joining forces with a leading animal remedy company, Deosan, as well as chemists from the University of Auckland, Prof Cook and co-lead investigator Dr Adam Heikal are heading up a team dedicated to finding new bio-active, sanitising molecules to help stop dairy herds developing mastitis in the first place, thereby reducing the farmers’ antibiotic needs.
Sanitisers for dairy cows are already widely used but the bioactive molecules they contain are also used extensively in hospitals across the country and around the world. To reduce the chances of resistance to these molecules arising on the farm, and spreading to the clinic it’s vital the new molecules, specifically for farm use, are discovered and developed. That forms one part of the final R, Replace.
The search for new antimicrobials is a never-ending one. As fast as new drugs are discovered, resistance starts to evolve. For this reason, the Cook lab invests heavily in screening large libraries of chemical compounds and natural products against problem bacteria, looking for new antimicrobial drug targets. However whole organisms, even those as small as a bacterial cell, are extremely complex. To reduce the levels of complexity, once a new antimicrobial target is identified, using either reverse genetics or a good old-fashioned experimental process of elimination, the target can be purified away from the organism and studied in greater detail. Three-dimensional structures can guide researchers to make more potent, more specific drugs. Recently, the Cook lab published the first bacterial structure of a protein long thought to be a drug target for tuberculosis treatment. The challenge now is to understand, at a molecular level, the enzyme’s inner workings and to ultimately inhibit its mode of action, leading to the design of a new antimicrobial drug.
The combination of all these research activities, as well as extensive international and industrial collaborations, help the Cook lab keep New Zealand at the forefront of antimicrobial discovery.
Check out some of the data about tuberculosis and vaccinations for whooping cough from the Figure.NZ team:
https://figure.nz/chart/0tUNDA6VMX0x6W5z
https://figure.nz/chart/AzwxPvVYJbh0lrcY-7fKh6eHZQCbjqKme
InfectedNZ data board: https://figure.nz/@InfectedNZ/public
About
Adam Heikal PhD is a former Research Fellow in the Department of Microbiology and Immunology, University of Otago. He continues to work in AMR and antimicrobial discovery at the School of Pharmacy, University of Oslo and remains in close collaboration with Prof Cook as co-Principal Investigator on projects related to the New Zealand Agritech sector. Follow Adam on LinkedIn.
What is InfectedNZ?
Hey, Aotearoa. It’s time we had a chat about infectious diseases and what we’re going to do about the looming antimicrobial armageddon. That’s why we’ve asked leading health, social and economic researchers, and people with personal stories, to help us get real about our vulnerability and discuss solutions. Follow their blogs right here at tepunhahamatatini.ac.nz and watch the conversation spread across social media with #infectedNZ.
Backing it all up, wherever possible, is data from the good folk at Figure.NZ. Their super duper charts are based on data sourced from public repositories, government departments, academics and corporations. Check out their #infectedNZ data board and sign-up to create your very own data board on any topic that floats your boat.

A different approach to developing new antibiotics
We are nearing a crisis point in our use (and, sadly, misuse) of antibiotics. Indeed, the World Health Organisation recently described humanity as being in “a race against time” to develop antibiotics against multi-drug resistant superbugs [i]. If we cannot find effective new antibiotics soon, we may be faced with a return to the 1920s pre-antibiotic era, where people routinely died of the most mundane things, like a scratch from a rose thorn while gardening.
A couple of important contributing factors have been the widespread prescription of antibiotics for patients with viral infections, and failure of patients to complete courses of antibiotics once they start feeling better. Because antibiotics don’t work against viruses, the first scenario does nothing to treat disease but does place all of our hundreds of species of natural gut microbes under a “selective pressure”, such that any that evolve the ability to resist the antibiotic will hang around while those around them are killed. Even though these microbes may actually be good for us, bacteria are unbelievably good at passing genes to one another, so new resistance genes may quickly find their way to dangerous disease-causing bacteria. The second scenario, though, provides an even more direct route to dangerous drug-resistant bugs. If we stop a course of antibiotics when only 99% of the disease-causing bacteria have been killed, the remaining 1% – which will include those most naturally resistant to the antibiotics – may come charging straight back at us.
The good news is, we have learned a lot over the past 70 years about how to better use antibiotics to slow the development of resistant bacteria. The bad news is that in that time we have burned our way through nearly all of the antibiotics discovered to date.
Dr Jeremy Owen and I lead a team at Victoria University that is taking two different approaches to try and get new antibiotics into the developmental pipeline. We are particularly interested in molecules known as ‘non-ribosomal peptides’ and ‘polyketides’. These molecules are commonly antibiotics that are made by bacteria to defend themselves against other microbes. They are built inside the bacteria by enzymes – highly specialised little nano-machines that link together to form an assembly line, with each section of the assembly line responsible for adding on a specific part of the antibiotic molecule in a Lego-like fashion (see illustration).

The enzymatic assembly lines that make non-ribosomal peptide and polyketide antibiotics are made up of discrete subunits that each play a precise role in recognising and joining different parts of the final product molecule, passing it down the line, and ultimately releasing it. Image credit: JVE Chan-Hyams, modified from DF Ackerley ‘Cracking the non-ribosomal code’ Cell Chem Biol 2016 23(5): 535-7.
One of our team’s approaches is to re-engineer the assembly line in the hope that we can build analogues of existing antibiotics that may get around the resistance mechanisms that disease-causing bacteria have evolved. We have developed approaches to swap out different sections (labelled 1-4 in the Illustration) of an assembly line that makes a particular molecule and replace them with other sections that cause a slightly different ‘Lego part’ to be added into the product molecule. This has been a really fascinating engineering problem, and we have learned a lot about how the assembly lines work, but this line of work is still in its infancy and for now remains a very difficult and inefficient way of making a new drug candidate.
Our more pragmatic approach has been to try and find previously undiscovered antibiotic molecules from nature. The majority of antibiotics in use today were discovered by growing bacteria isolated from different soils around the globe, and testing the different molecules they naturally secrete. From the 1940s to 1960s this was a highly productive approach, but thereafter researchers struggled to find anything new – the same sets of molecules just kept cropping up time and time again. In recent times we have realised that only a very small proportion of soil bacteria – under one percent! – can be grown effectively outside of their natural environment. It is therefore extremely likely that the remaining 99% produce some very effective antibiotics that we have previously been unable to access.
To get around the problem that we cannot presently grow most soil bacteria in the lab, we instead go straight to their DNA, purified directly from the soil. We have developed and optimised several different strategies to ‘fish out’ clusters of gene that encode the types of assembly line pictured in the Illustration. These effectively act as blueprints that tell a cell how to make one particular antibiotic. Taking advantage of the fact that bacteria are so good at swapping bits of DNA, we and others have shown that you can transfer these blueprints to a new host – a bacterium that we can grow in the lab – and a surprising amount of the time it will gain the ability to produce a new antibiotic! Luckily for us, most antibiotic gene clusters not only encode the assembly line needed to make an antibiotic, but also a means for defending the host cell against any toxic effects.
This is still a new line of research in New Zealand, but before he returned home in 2015, Jeremy successfully used similar approaches at The Rockefeller University in New York. There he discovered, produced, and tested a number of previously unknown molecules, including novel antibiotics and compounds with anti-cancer potential (see paper one and two on the Proceedings of the National Academy of Sciences USA. We already have indications that NZ soils are rich in equally promising new drug candidates, and are working hard to pull some of those out. However, rigorous testing standards mean that even if we do find new antibiotics that work well in people, it will likely be at least ten years before any of these enter clinical use. Nevertheless, it is critical to keep the discovery pipelines flowing if we are to avert the crisis foreseen by the World Health Organisation and numerous other medical organisations around the globe.
[i] Bulletin of the World Health Organization 2011;89:88–89. doi:10.2471/BLT.11.030211
Associate Professor David Ackerley and colleagues from Victoria University Wellington recently featured on the cover of a leading international journal with an image of bacteria grown in the shape of New Zealand. The cover related to their work investigating the potential of engineering improved antibiotics.
About
Associate Professor David Ackerley is from the School of Biological Sciences at Victoria University Wellington. A major theme of his research is directed evolution, artificial acceleration of rates of mutation and recombination in genes and genomes, and selection of variants with improved qualities. David is also an Associate Investigator for the Maurice Wilkins Centre of Molecular Biodiscovery.
What is InfectedNZ?
Hey, Aotearoa. It’s time we had a chat about infectious diseases and what we’re going to do about the looming antimicrobial armageddon. That’s why we’ve asked leading health, social and economic researchers, and people with personal stories, to help us get real about our vulnerability and discuss solutions. Follow their blogs right here at tepunhahamatatini.ac.nz and watch the conversation spread across social media with #infectedNZ.
Backing it all up, wherever possible, is data from the good folk at Figure.NZ. Their super duper charts are based on data sourced from public repositories, government departments, academics and corporations. Check out their #infectedNZ data board and sign-up to create your very own data board on any topic that floats your boat.
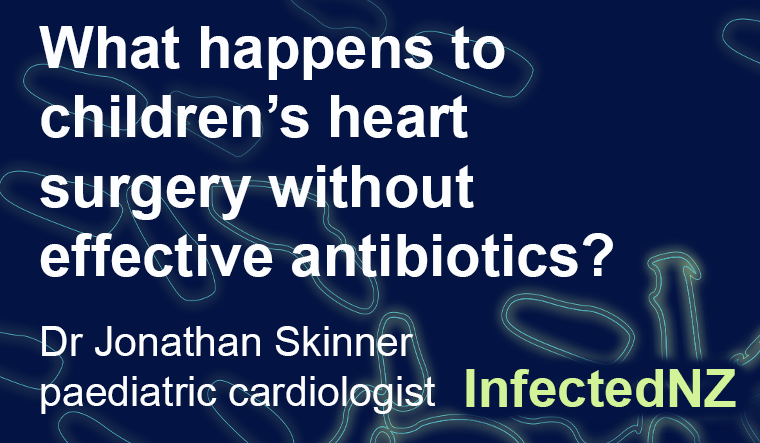
What happens to children’s heart surgery without effective antibiotics?
Heart defects are collectively the commonest abnormality in newborn babies. About one infant in every 150 has some form of defect; a hole in the heart, or parts of the heart underdeveloped or in the wrong place. Four hundred children every year undergo heart surgery in New Zealand at the Starship Children’s hospital, and another 250 undergo keyhole (cardiac catheter) interventions. Currently over 97% survive their operation, including the most complicated cases and most severely ill infants. Most leave the hospital well, with a neat “zipper” scar down the front of their chest which will gradually fade to a pale white line.
The very first heart operation in the world, in 1895 in Norway, was on a 24 year old man. He survived the surgery, but died several days later from overwhelming infection. It was relatively simple surgery but there were no antibiotics at that time.
These days, surgeons introduce goretex to patch holes, plastic and metal valves to replace faulty valves, and electrical pacemakers to keep a stable rhythm. Operations are done in state-of-the-art operating rooms with controlled air flow, and great efforts are taken to keep the operating field sterile. Even so, internationally about one in five patients (20%) get an infection early after the surgery, most commonly in the lungs or in the surgical wound. 3% overall get an immediately life threatening infection such as one around the heart, or in the blood. When treated with a course of antibiotics, they can fully expect to recover. Today, it is rare for a child to die from such post-surgical infection, even in severe cases.
So what would happen without antibiotics? Modern children’s heart surgery has never been performed without the availability of antibiotics, so, in truth, it is impossible to know for sure how many infants and children would die or suffer prolonged illness or disfigurement without them. We can be sure that 3% will die from their severe infections. Given that around 20% will get an infection, we can guess that the post-operative ward would be an entirely different place. It would be a place of rampant bacterial infection, pus draining from open wounds, chest walls never healing properly, and some children staying for many weeks or months. Infected children and their visiting family would have to be isolated from other children. And it would be a place of death. The vulnerable very sick newborns would succumb in large numbers. And it will be a painful and often foul-smelling death.
The spectre of infection would hang over every procedure. Hospitals in general will be a place of death and suffering more than hope and recovery. So the threshold for doing an operation will go up. Parents will naturally be too scared to allow their child to undergo what today would be a routine procedure. Children therefore will suffer the consequences of their heart problem for longer; suffering shortness of breath, poor energy and unable to take part in sport. Some would be unable even to get to school. Infants in heart failure would not gain weight and may die from chest infections.
I am also imagining myself in a world without antibiotics. I could be confined to a wheel chair, because my hip surgery would have been inadvisable given the high risk of infection. That nasty infected boil I once had from a mosquito bite would possibly have disfigured and damaged much of my right hand, a hand I need to perform heart procedures.
I hope that what we heart specialists do now for the children we care for represents merely the dawn of even greater things. But if we don’t get more antibiotics, and use them wisely, it may be that future parents and their children will be longing for the past golden age of children’s heart surgery and intervention; 2016.
About
Jonathan Skinner is a paediatric cardiologist/electrophysiologist and Auckalnd’s Starship Children’s Hospital and an Honorary Professor of Paediatrics Child and Youth Health at the University of Auckland.
What is InfectedNZ?
Hey, Aotearoa. It’s time we had a chat about infectious diseases and what we’re going to do about the looming antimicrobial armageddon. That’s why we’ve asked leading health, social and economic researchers, and people with personal stories, to help us get real about our vulnerability and discuss solutions. Follow their blogs right here at tepunhahamatatini.ac.nz and watch the conversation spread across social media with #infectedNZ.
Backing it all up, wherever possible, is data from the good folk at Figure.NZ. Their super duper charts are based on data sourced from public repositories, government departments, academics and corporations. Check out their #infectedNZ data board and sign-up to create your very own data board on any topic that floats your boat.

A future without antibiotics
Last week was World Antibiotic Awareness week, an initiative of the World Health Organization (WHO) to raise awareness and understanding of antimicrobial resistance. To follow-up, here at Te Pūnaha Matatini we are having a week-long conversation about the health, social, economic, and environmental impacts of infectious diseases in Aotearoa New Zealand. In this post, I want to touch on what antimicrobial resistance is, and what a future without antimicrobial medicines could look like.
What is antimicrobial resistance and what is causing it?
Antimicrobials are chemicals that kill or stop the growth of microbes. But as microbes is the generic term for a multitude of life forms which differ in their genetic make-up, life-styles and habitats, so antimicrobials can be divided into different categories depending on what they target. Some antimicrobials work against all microbes, but others are more specific. Antivirals only work against particular viruses, antifungals only work against particular fungi and antibiotics only work against particular bacteria.
Antimicrobial resistance is when microbes develop the ability to stop antimicrobials from affecting them. As most microbes replicate themselves and their genetic material fairly rapidly (some can divide in just a few minutes), and they can grow to large numbers (easily reaching population sizes in the billions if they have the right conditions), there are plenty of opportunities for resistant mutants to arise purely by chance. These mutants can then grow quite happily in the presence of the antimicrobial. This happens wherever microbes encounter antimicrobials – in human and veterinary medicine, in agriculture, but also in sewage systems and out in the wider environment. More worryingly is when microbes gain the ability to share resistance between each other on mobile bits of genetic material. Then they don’t even need to be in the presence of the antimicrobial agent – they just need to meet the right kind of resistant microbe!
A major factor in the development of resistance is the misuse and overuse of antimicrobials. So being used when they aren’t needed, or not being used correctly. Another worry is the use of similar antimicrobials in human medicine and in agriculture. For example, a fungus commonly found in soil has become resistant to the antifungal pesticides used in gardening and agriculture. Because similar antifungals are used in human medicine, these resistant fungi are now able to cause almost untreatable infections in some vulnerable hospital patients. And these patients can become infected just by being in a bed next to an open window that looks out onto a garden!
Is antimicrobial resistance something we should be worried about?
Yes. Experts predict that within the next decade we will run out of antimicrobial medicines to treat many common infections. Part of the reason we are in this position is that most of the pharmaceutical industry pulled out of antimicrobial research decades ago, so the medicine cupboard is basically empty. Similarly, the vast majority of government and charity funding around the world has gone on researching non-communicable diseases. Any new antimicrobial compound discovered today could take a decade of development and testi before it would be available for doctors to use. The situation is a catastrophe on a par with global warming.
What are the most concerning examples of resistant strains of infectious diseases?
The major resistant bacteria that are circulating around the world are extended-spectrum beta-lactamase (ESBL) expressing strains of Escherichia coli and Klebsiella pneumoniae, which are of particular concern in hospitals, and some strains of Mycobacterium tuberculosis which causes the lung disease TB. There are an increasing number of strains of these bacteria that are sensitive to just one or two antibiotics, and some strains that are pretty much untreatable. Another resistant organism of growing concern globally is Neisseria gonorrhoeae which causes gonorrhoea. While most men with gonorrhoea will have symptoms when they have the disease, half of women can be asymptomatic so won’t know they are infected. Importantly, untreated gonorrhoea can lead to infertility.
What’s happening in New Zealand?
We wanted to show you the data, but can’t. It is publicly available on the web but Figure.NZ were denied permission to turn it into nice charts for you to see. What we can say is that the extremely resistant strains of E. coli, K. pneumoniae and M. tuberculosis we see here are mainly coming into New Zealand from countries like India, China, and those in south-east Asia. This is going to be an area to watch, especially given the importance of countries like China for trade and tourism in New Zealand.
N. gonorrhoeae is also one for us to watch as highly resistant strains have been reported in Australia. In New Zealand, gonorrhoea is not a notifiable disease so the only data we have is based on the voluntary provision of the numbers of diagnosed cases from laboratories and sexual health and family planning clinics. In 2014, that number was 3,038, with 977 of these cases in young people under the age of 19. Less than half of sexually active young people report using condoms which would protect them from infection. If we end up with a completely untreatable strain of N. gonorrhoeae taking hold in New Zealand this could have a huge impact on our future fertility.
The last organism of concern here is Methicillin Resistant Staphylococcus aureus (also known as MRSA) which is very much a problem of our own making. Over the last few years there has been a huge increase in the number of skin and soft tissue infections caused by S. aureus in New Zealand (1). Alongside this, there has been a huge increase in prescriptions for a topical antibiotic called fusidic acid. As a consequence, one of the major clones of S. aureus now causing disease in New Zealand is an MRSA clone which is resistant to fusidic acid (2).
Major misconceptions about antibiotics
There are a number of major misconceptions about antibiotics. Lots of people don’t know that bacteria and viruses are very different life-forms. This means that antivirals don’t work on bacteria, and antibiotics don’t work on viruses. In countries where antibiotics are available without prescription, many people confuse antibiotics with pain-killers, so will take antibiotics for things like a headache!
Another common misconception is that it is us that become resistant to antibiotics, rather than the microbes. Perhaps this is a misunderstanding between how antibiotics work (by killing the bacteria directly), versus what happens when we are vaccinated (our immune system is primed to recognise and fight off the invader).
But the biggest misconception is that people who don’t take antibiotics, or who take them very rarely, won’t be affected by antibiotic resistant bacteria – that the antibiotics will still work for them. It doesn’t matter if you’ve never had a course of antibiotics, or if you’ve had several, it all depends on the bacteria you get infected with. Similarly, healthy people who have never had a course of antibiotics could still have antibiotic-resistant superbugs living happily up their noses or in their guts. They can easily spread from person to person, or can be picked up while travelling overseas.
What does a future without antimicrobials look like?
A future without antimicrobials will affect us all; rich and poor, young and old. In a world without antimicrobials, previously treatable infections will once again become deadly, or may require amputation to stop them in their tracks. Because antimicrobials are also used to prevent infection in vulnerable people, it will also become life threateningly risky to do routine operations like caesarean sections and joint replacements, and treatments like chemotherapy for cancer.
Margaret Chan, Director-General of the World Health Organization, called antimicrobial resistance “…the end of modern medicine as we know it”. In a series of reports commissioned by the UK’s former Prime Minister David Cameron, economist Sir Jim O’Neill has estimated that without urgent action, antimicrobial resistance will kill 10 million people a year by 2050, more than will die from cancer. O’Neill has also put an economic cost on the issue, estimating that inaction will cost the world’s economy 100 trillion USD by 2050.
What should we be doing to combat antimicrobial resistance?
Combatting antimicrobial resistance requires a global effort to stop the overuse and misuse of antimicrobials in human and veterinary medicine, and in agriculture. It requires governments, philanthropists, charities and industry to invest serious money into antimicrobial discovery and development and research into new ways to combat infectious diseases. We also need quicker and better ways to diagnose infectious diseases so that patients can receive the right treatment as soon as possible.
Equally important is that we have a national conversation about how we all, the public, health workers, policymakers and the agricultural sector, can contribute to solving this global crisis from right here in New Zealand. I hope you’ll participate in this important discussion. Follow #infectedNZ on Twitter or Facebook, or leave a comment below.
References:
- Williamson DA, Zhang J, Ritchie SR, Roberts SA, Fraser JD, Baker MG (2014). Staphylococcus aureus infections in New Zealand, 2000-2011.Emerg Infect Dis. 2014 Jul;20(7):1156-61. doi: 10.3201/eid2007.131923.
- Williamson DA, Monecke S, Heffernan H, Ritchie SR, Roberts SA, Upton A, Thomas MG, Fraser JD (2014). High usage of topical fusidic acid and rapid clonal expansion of fusidic acid-resistant Staphylococcus aureus: a cautionary tale. Clin Infect Dis. 2014 Nov 15;59(10):1451-4. doi: 10.1093/cid/ciu658.
About:
Dr Siouxsie Wiles is Deputy Director of Te Pūnaha Matatini. She describes herself as a microbiologist and bioluminescence enthusiast. Head of the Bioluminescent Superbugs Lab at the University of Auckland, Siouxsie combines her twin passions to understand infectious diseases.
What is InfectedNZ?
Hey, Aotearoa. It’s time we had a chat about infectious diseases and what we’re going to do about the looming antimicrobial armageddon. That’s why we’ve asked leading health, social and economic researchers, and people with personal stories, to help us get real about our vulnerability and discuss solutions. Follow their blogs right here at tepunhahamatatini.ac.nz and watch the conversation spread across social media with #infectedNZ.
Backing it all up, wherever possible, is data from the good folk at Figure.NZ. Their super duper charts are based on data sourced from public repositories, government departments, academics and corporations. Check out their #infectedNZ data board and sign-up to create your very own data board on any topic that floats your boat.

Antibiotics – invented by fungi, harnessed by humans
The fungi are a vast and distinctive kingdom of organisms that make up a significant component of most land ecosystems, intimately linked with bacteria, plants, and animals. Fungi cannot make their own food, so they live on or within their food, be that dead wood or leaves, overmature fruit, or a living plant or animal. In these habitats, a fungus competes with other life forms including other fungi and also bacteria. Some species of fungi have adapted to growth in hostile habitats, while others developed biochemical defences to fight off competitors. The awareness and application of these fungal defences has revolutionised modern medicine.
The idea that a substance could be used as a ‘magic bullet’ to target disease causing organisms dates from the late 19th Century. In 1909 Nobel prize winner Paul Ehrlich and his team effectively began the science of drug discovery when they developed the organic arsenic compound Salvarsan to treat syphilis. But it wasn’t until the chance discovery of antibacterial action by fungi that antibiotics became one of humanity’s major medical advances.
Alexander Fleming is credited with the discovery and naming of penicillin. In 1928 he returned from holiday to St Mary’s Hospital, London, to find previously living cultures of the bacterium Staphylococcus aureus contaminated and killed by a mould fungus Penicillium notatum. Fleming kept the fungal culture but wasn’t able to purify and stabilise penicillin. This achievement was left to Florey, Chain & Heatley at Oxford University. Subsequent researchers in the UK and USA perfected the production by fermentation and later chemical synthesis, enabling a revolution in medical intervention to counter previously lethal conditions such as pneumonia, septicaemia, and gangrene. Before antibiotics, bacterial infection of a skin wound often led to growth of bacteria that overwhelmed the body’s immune system. In World War 1, for example, more than 1 in 10 soldiers injured in battle died of infected wounds.
The life-saving success of penicillin led to intensive research, especially until 1970, to discover new antimicrobial compounds produced by fungi and filamentous bacteria. This gave us antibiotics such as cephalosporin produced from Cephalosporium; neomycin, tetracycline and streptomycin (to initially control tuberculosis (TB)) from Streptomyces (Actinobacteria), and antifungals such as griseofulvin from Penicillium griseofulvin.
Since 1970, there have been very few new types of antibiotics discovered. At the same time, disease-causing bacteria have evolved to become resistant to our medicines, making currently available antibiotics less and less effective. Even worse, bacteria evolve antibiotic resistance much faster than the pace of new antibiotic discovery. Unfortunately, the major pharmaceutical companies have diverted research funds to more profitable drug development for chronic diseases rather than for bacterial infections. There’s an urgent need to develop new responses to overcome antibiotic-resistant superbugs, and so avoid a return to pre-penicillin days when an infected cut could be lethal.
This has been the motivation for a number of new research initiatives seeking novel antibiotics and other means to control bacterial infection. New Zealand’s national living culture collection of fungi and plant-associated bacteria is a research resource for national benefit, managed by Landcare Research. Bevan Weir, as Curator of the International Collection of Microorganisms from Plants (ICMP), is collaborating with Siouxsie Wiles and her University of Auckland postgraduate students seeking new antibiotic compounds from among the 20,000 cultures of fungi and bacteria. The New Zealand collection began in the 1950s, and includes many native species that have not previously been investigated for antibiotic production.
References
Livermore DM 2011. Discovery research: the scientific challenge of finding new antibiotics. J. Antimicrob. Chemother. 66: 1941–1944.
Moore D, Robson GD, Trinci PJ 2011. 21st Century Guidebook to Fungi. Cambridge University Press, Cambridge.
Overbye KM, Barrett JF 2005. Antibiotics: where did we go wrong? Drug Discovery Today 10: 45-52.
About
Peter Buchanan works for Landcare Research as Science Team Leader for a group of 30 science staff researching New Zealand’s most diverse groups of terrestrial organisms – the fungi, bacteria, invertebrates, and plants. Landcare Research is custodian of five national biological collections including the NZ Fungal Collection (PDD) and Culture Collection of Fungi and Bacteria (ICMP). Peter’s research interests are in fungal conservation, wood decay fungi, applied uses of fungi, and science education.
What is InfectedNZ?
Hey, Aotearoa. It’s time we had a chat about infectious diseases and what we’re going to do about the looming antimicrobial armageddon. That’s why we’ve asked leading health, social and economic researchers, and people with personal stories, to help us get real about our vulnerability and discuss solutions. Follow their blogs right here at tepunhahamatatini.ac.nz and watch the conversation spread across social media with #infectedNZ.
Backing it all up, wherever possible, is data from the good folk at Figure.NZ. Their super duper charts are based on data sourced from public repositories, government departments, academics and corporations. Check out their #infectedNZ data board and sign-up to create your very own data board on any topic that floats your boat.

Antimicrobial use in animals: where do we stand?
One thing is clearer than ever: the wellbeing of animals, humans and the environment is inseparable and, on a global scale, no issue represents this complex relationship better than the rise of antimicrobial resistance.
The medical discovery that revolutionised our ability to treat disease in humans and animals is becoming its own worst enemy. The pressure is on the veterinary and medical communities internationally to find solutions. It’s a ‘One Health’ issue in its purest form.
Last year the New Zealand Veterinary Association (NZVA) tackled the issue of antimicrobial resistance (AMR) head-on. It challenged the veterinary profession, farmers, pet owners, government and industry to rethink the use of antibiotics for animals. It set an aspirational vision that “by 2030 New Zealand Inc. will not need antibiotics for the maintenance of animal health and wellness”.
It’s a big ask, and not necessarily a vision that everyone immediately supports. It’s also a carefully worded statement acknowledging that while the science is complex and sometimes contradictory, everyone in essence agrees that the more we use these valuable medicines, the more likely resistance will be selected for. It also acknowledges that the issue the veterinary profession must address most urgently is to reduce, refine, and replace the use of antimicrobials for maintenance (prophylactic and metaphylactic use) while maintaining their therapeutic use to protect the wellbeing of our animals.
The science of AMR is challenging, challenged, and still emerging, which means the issue is poorly understood by most people. What is poorly understood by even the scientific community is that, when measured against the estimated biomass of animals in the country, New Zealand is already the third lowest user of antibiotics for animals in the OECD.
Moreover, data published this year showed that while the average use of antibiotics in animals is 9.4mg active ingredient/kg biomass, the human use is 121mg active ingredient/kg biomass, 12.9 times higher and the 16th highest of the countries compared. This is a simple indicator of comparative use in what we all acknowledge to be a scientifically complex issue (we must, for example, also consider the critically important nature of the actives used in veterinary medicine and that many of these actives are for veterinary use only), but it reflects how effective regulation and control by veterinarians over prescribing practices is maintaining New Zealand in a position of world leadership in the stewardship of these vital medicines.
Dr Stephen Page, an internationally recognised veterinary pharmacologist, has proposed that the previous Hypercene era of profligate antimicrobial use is moving in to the Hypocene, era of over restraint. This is an inevitable step, he says, before the human and veterinary professions reach the Eucene, the era of appropriate use.
This may well be. In order to navigate to the Eucene, we must ensure that scientific evidence is used as the basis of any regulatory or stewardship changes made to address society’s growing concerns around antimicrobial usage. We must also ensure that access to these vital medicines is maintained to responsibly treat bacterial disease, for the wellbeing of animals, humans, and the environment.
Reference
Hillerton JE, Irvine CR, Bryan MA, Scott D, Merchant SC (2016). Use of antimicrobials for animals in New Zealand, and in comparison with other countries. New Zealand Veterinary Journal, Forthcoming articles, pp 1-7, Mar 2016.
About
Callum Irvine is an experienced vet and the Head of Veterinary Services for the New Zealand Veterinary Association (NZVA) where he champions veterinary leadership and advocates on behalf of the veterinary profession. Through his role he promotes science based policy development across all areas of veterinary science.
What is InfectedNZ?
Hey, Aotearoa. It’s time we had a chat about infectious diseases and what we’re going to do about the looming antimicrobial armageddon. That’s why we’ve asked leading health, social and economic researchers, and people with personal stories, to help us get real about our vulnerability and discuss solutions. Follow their blogs right here at tepunhahamatatini.ac.nz and watch the conversation spread across social media with #infectedNZ.
Backing it all up, wherever possible, is data from the good folk at Figure.NZ. Their super duper charts are based on data sourced from public repositories, government departments, academics and corporations. Check out their #infectedNZ data board and sign-up to create your very own data board on any topic that floats your boat.

Dolphins and infectious diseases: a story of toxoplasmosis
Toxoplasmosis is an infectious disease caused by parasite, Toxoplasma gondii. It is an important cause of disease in a wide range of species, including humans, domestic animals and wildlife species.
Cats are the only known source of the infectious stage (the oocyst) of the parasite, and can shed millions of these microscopic cysts in their faeces. Oocysts are extremely resistant in the environment, and infect a new host when they are accidentally swallowed in food or water that has been contaminated by cat faeces.
After they’ve been ingested, oocysts can spread throughout the body in the blood stream, causing clinical disease, or encyst and become latent in body tissues. As well as accidental ingestion, hosts can also be infected by eating encysted Toxoplasma organisms in tissues of prey or food items, or by transfer across the placenta to a fetus when a pregnant host becomes infected.
In humans, disease can be either mild and flu-like, or more severe and debilitating, especially in newborn children and in patients who are immunosuppressed. Some recent studies have also suggested a link between Toxoplasma infection and psychiatric disorders.
For many years toxoplasmosis was considered to be mainly a terrestrial disease, but more recently increasing numbers of water-borne infections have been recognised. Human infections have occurred overseas following contamination of water sources with faeces from wild large cats, and in several countries coastal marine mammals such as sea otters have been found to be infected.
Our research group is contracted by the Department of Conservation to investigate deaths in several marine mammal species, and between 2007 and 2016 we diagnosed fatal toxoplasmosis in eight endangered New Zealand Hector’s dolphins, including two critically endangered Maui dolphins. All of these infections were caused by a specific strain (genotype) of Toxoplasma, which appears to be identical to a genotype we have found in wild birds.
We believe that oocysts reach the New Zealand coast in freshwater runoff contaminated by cat faeces. The genotype we have found in dolphins and birds could be either extremely common in this country, or cause particularly severe infections in these species. To investigate this disease further we are conducting a series of studies looking at the frequency of infection and the genotypes present in domestic and feral cats in different parts of New Zealand, and possible transmission pathways for oocyst contamination of waterways. We are particularly interested in identifying risk factors for contamination of the marine environment, including high volumes of rainfall and proximity to urban settlements or large cat populations.
Toxoplasmosis isn’t the only infectious disease that we’ve found in Hector’s dolphins. One of the biggest impacts on the species is believed to be accidental capture in fishing nets, but our work at Massey shows that disease is an additional factor contributing to Hector’s dolphin deaths.
In one of our studies, 13/49 (27%) of the dolphins examined had died due to an infectious disease, either bacterial, fungal or parasitic. We’ve made similar findings in investigations of New Zealand sea lion deaths.
While it appears as though infectious disease is on the increase in our marine mammals, it is difficult to be absolutely sure about this, since our ability to diagnose these infections has also improved over the years.
Our findings show how important it is to investigate infectious disease in wildlife species, including going back to re-examine stored samples using newer techniques. As we continue to do this we will begin to build a better picture of the impacts we are having on our natural world, and the effects these changes will have on our own health and that of our environment.
About
Dr Wendi Roe is a veterinary pathologist and marine mammal researcher based at Wildbase, in the Veterinary School at Massey University, Palmerston North.
She began her veterinary career with 10 years in “normal” practice, working with cats, dogs, and farm species, before going back to university in 2000 to train in pathology and work with marine mammals. Current research carried out by Wendi and her group focusses on causes of disease and death in endangered New Zealand marine mammals.
Find out more about toxoplasmosis in a blog by Siouxsie Wiles.
What is InfectedNZ?
Hey, Aotearoa. It’s time we had a chat about infectious diseases and what we’re going to do about the looming antimicrobial armageddon. That’s why we’ve asked leading health, social and economic researchers, and people with personal stories, to help us get real about our vulnerability and discuss solutions. Follow their blogs right here at tepunhahamatatini.ac.nz and watch the conversation spread across social media with #infectedNZ.
Backing it all up, wherever possible, is data from the good folk at Figure.NZ. Their super duper charts are based on data sourced from public repositories, government departments, academics and corporations. Check out their #infectedNZ data board and sign-up to create your very own data board on any topic that floats your boat.