Investigators' Blog

If only there had been a vaccine
“Only clean water and antibiotics have had an impact on childhood death and disease that is equal to that of vaccines” – World Health Organization (WHO).
In the 20th century, when most of you reading this were born, nearly 1.7 billion people died from infectious diseases.
Most of the diseases in this sobering infographic are now vaccine preventable through the creative collective brilliance of many scientists. Smallpox, influenza, diarrhoea (rotavirus), whooping cough, meningitis, tetanus, Hepatitis B, rabies, and measles are the diseases responsible for carrying off most of those 1.7 billion people.
While the First World War raged for over four years and took the lives of 16,697 New Zealanders, in 1918 influenza took about half as many in the space of just two months (8600 New Zealanders died).
So how many lives have vaccines saved? I have never been able to find an overall total, but one bunch of scientists (ScienceHeros.com) estimate that Edward Jenner alone was responsible for saving 530 million lives. In other words, that is how many deaths from smallpox are estimated to have been prevented. I thought that sounded like a lot before I remembered that the estimate for the 20th century alone is about 300-500 million so maybe this is actually a conservative estimate although I have not been able to find any convincing estimates on this. Imagine inventing something that saved half a billion lives!
That made me think of another major killer – measles.
Already this century the WHO has estimated that measles vaccine alone has saved over 17 million lives. A couple of doses at a cost of about $NZ1 works a treat. And if you think measles never really hurt any Kiwis then I suggest you have a look at this New Zealand timeline and contemplate how small the population was during these years. In 1900 we numbered little more than 700,000
The man responsible for this impressive achievement is Maurice Hilleman who developed a measles vaccine which is estimated to have saved 118 million lives since the 1960s. Every country in the world uses measles vaccine and the disease is on track for global elimination. That is one hell of a legacy, but Hilleman didn’t finish there. He developed over 40 vaccines including those against influenza, mumps, hepatitis A and B, chickenpox, meningococcal, and Haemophilus influenza type B, How many more lives do these account for?
The infectious landscape in NZ is very different today than it was. Once upon a time, before air travel, if someone infectious got on a boat they had either died or recovered by the time they arrived here some 135 days later. New Zealand’s isolation made her a natural quarantine zone.
But in the end this did not stop the importation of infectious diseases and as more people came so did their diseases. The first documented outbreak of a now vaccine preventable disease was an influenza outbreak in 1817-20. From then on epidemics occurred regularly, decimating family sizes and disproportionality affecting Māori to the point where the population had halved by the late 1800s.
If one examines the chronology of events major enough to affect the health and size and life expectancy of the New Zealand population since 1850, it is striking how many of these are either infectious epidemics (negative effect) or the introduction of a vaccine (positive effect). (Editor’s note: the Statistics New Zealand website may be down due to 14 November earthquake).
The chronology starts with an influenza epidemic in 1852-3. In 1873 there was a notable pertussis epidemic with 356 deaths noted and the very next year a measles epidemic taking 344 children along with diphtheria killing 481 in the same year. These four diseases go on to appear as major events every few years. Today they are all preventable but still not curable.
Watch: Everything you want to know about immunisation but were afraid to ask.
A timeline of epidemics affecting New Zealand shows almost all of the most dangerous diseases (those that carried off the most people) are now be vaccine preventable. These diseases in order of first appearance in NZ were:
- 1817 Influenza
- 1835 Measles
- 1863 Scarlet fever, aka strep throat
- 1872 Diphtheria
- 1872 Smallpox (only the once in 1872)
- 1873 Pertussis
- 1874 Typhoid
- 1875 TB
- 1900 Plague
- 1916 Polio
- 1921 Meningitis (probably meningococcal disease)
- 1939 Rubella
- 1971 Hepatitis A
- 1983 HIV/AIDS
- 1997 Campylobacteriosis
- 2009 H1N1 swine flu
Most of these (with the exception of smallpox) caused repeated epidemics every 3 – 5 years until their respective vaccines were introduced. The bold ones are vaccine preventable to a greater or lesser extent.
While New Zealand never really suffered from smallpox, in part thanks to isolation, it was not until the introduction of the first national immunisation programme that sickness and death from these infectious stopped.
Enter vaccination
In 1926 the first vaccine was introduced into New Zealand. Diphtheria vaccine became available to schools and orphanages, but it was not until 1941 that it became routine for all children under seven years old. Below is what happened next:

Number of cases of diphtheria and diphtheria mortality, 1916–2013. Source: Ministry of Health and the Institute of Environmental Science and Research.
The next cab off the vaccine rank to be introduced into New Zealand was Tetanus after 1940, however it was not until 1958 this became routine.
At this time we also began vaccinating against pertussis and the mortality rate plunged.

Numbers of cases of poliomyelitis, 1915–2013. Source: Ministry of Health and the Institute of Environmental Science and Research
Polio vaccine arrived in 1956 and shortly after the disease was eliminated in New Zealand.
Today
We have controlled these diseases through an effective immunisation programme which now reaches more of New Zealand’s children than ever before. In 1991 only about 56% of New Zealand children were fully vaccinated. This was a national shame, second from the bottom of all the OECD countries. Today we are awesome with around 94% of New Zealand children fully vaccinated against 11 diseases, soon to be 12. This puts us near the top of the OECD countries.
Twelve diseases we protect NZ children against before they are two years of age are:
- Diphtheria
- Tetanus
- Pertussis
- Polio
- Haemophilus influenza type B (a cause of meningitis)
- Hepatitis B
- Measles
- Mumps
- Rubella
- Pneumococcal disease
- Rotavirus
- Varicella (chickenpox) from 2017

Data for graphs are based on frequency of epidemics noted in the NZ historical records and the number of deaths noted in epidemics. The number of cases has been extrapolated by the estimates of mortality in the pre-vaccine era (JAMA 2007;298(18):2155-2163)
But
In New Zealand, vaccines have not been universally embraced. People have forgotten what these diseases are and what they did to families.
Today myths about vaccines circulate widely on social media where opponents actively seek to discredit the vaccination programme. While falsehood flies around the world on Twitter in one second the truth can have a hard time catching up, and by then the damage has been done. Confidence in life-saving vaccines has been shattered through deceit.
The evolution of a vaccine programme is predicable. The Chen graph below may be old now, but it sums up the evolution of vaccine programmes beautifully. First there is disease. A vaccine comes along and the disease declines. People start freaking out about (usually perceived not real) adverse events and lose confidence in the vaccine. Coverage declines, disease comes back. People realise disease is bad and they should never have stopped vaccinating, coverage improves. Disease declines again.
I wonder what the families who watched their children die from these diseases would think about those that actively seek to prevent people immunising their children? An opportunity they never had.
Vaccines have changed people’s perception of what a normal life expectancy is. Today the single biggest threat to preventing these, and many more diseases, is a lack of commitment to and a lack of confidence in vaccines. Many people perceive vaccines to carry a much greater risk than they do. Despite the fact that vaccines are one of the safest public health interventions ever developed this is not always the perception.
Check out more data about vaccines and hospitalisations from the Figure.NZ team:
https://figure.nz/@InfectedNZ/public
https://figure.nz/chart/AzwxPvVYJbh0lrcY-ss9cb1hXRe2y2C4x
https://figure.nz/chart/bn2ytKLMXjW0wUIk
About
Helen Petousis-Harris is a vaccinologist. Her background is predominantly biological sciences, and she did her PhD in vaccinology. She has an appointment as a Senior Lecturer in the Department of General Practice and Primary Health Care at the University of Auckland’s Faculty of Medicine and Health Sciences. She and has worked at the Immunisation Advisory Centre at the University of Auckland since 1998 where she has developed a passion for all things vaccine. Current favourite diseases are pertussis, pneumococcal disease, measles, meningococcal, HPV and gonorrhoea. Could be persuaded to turn attention to shingles.
What is InfectedNZ?
Hey, Aotearoa. It’s time we had a chat about infectious diseases and what we’re going to do about the looming antimicrobial armageddon. That’s why we’ve asked leading health, social and economic researchers, and people with personal stories, to help us get real about our vulnerability and discuss solutions. Follow their blogs right here at tepunhahamatatini.ac.nz and watch the conversation spread across social media with #infectedNZ.
Backing it all up, wherever possible, is data from the good folk at Figure.NZ. Their super duper charts are based on data sourced from public repositories, government departments, academics and corporations. Check out their #infectedNZ data board and sign-up to create your very own data board on any topic that floats your boat.

‘Gross us out Miss’: talking about chlamydia and other STIs with young people
Chlamydia is not a very sexy topic… it should be though, because it’s caught by having sex. It’s surprising how many young people don’t know how common chlamydia is. It’s easy to treat but it’s so much easier to prevent by using condoms when having oral, anal, or vaginal sex.
I’m a Nurse Practitioner for children and young people and also a Senior Lecturer at the University of Auckland. I work in a general practice clinic where we also provide health services for young people by going into three high schools.
Recently I’ve been asking young people about the best way to get information about STIs out to their age group. Chlamydia is a big problem for young people in New Zealand. In 2013 we tested 246 people between 15 and 18 years old who were seeking sexual health care for chlamydia. We found that 36% were positive. What people don’t realise is that chlamydia rarely causes any unpleasant symptoms, but it can have a lifelong consequence of infertility. So when you want to have a baby you can’t because your tubes (both male and female) are clogged up from past chlamydia infections.
Chlamydia is most commonly found in young people aged 15 – 29 years. Young women have higher rates than young men, but that may be due to women getting tested more frequently. What is most concerning is that young Māori women have rates 2-3 times higher than NZ Europeans.
“Social media like on Facebook, Instagram, Twitter and stuff” would be the best way to spread the word, said one group of 16 – 18 year old girls I asked (amidst chatter about whether you could get pregnant if you were the girl on top).
“You should start a movement or like have a campaign,” was another suggestion. They also said that they should be more educated about it and that it should be compulsory for Years 9 to 13. “They do it in intermediate [learn about sexual health] but that’s when they’re like really immature and they don’t listen.”
When asked what would help them learn about STIs, the girls thought that they should be “grossed out as much as you can” by showing them horrible pictures of infections and by getting testimonials from people who’ve had an STI. Scare tactics work, they said, because people don’t believe it will happen to them. “Come to the schools and talk to us in assemblies and put posters up with pictures, not heaps and heaps of words,” were a couple of suggestions. “Get on to Facebook to start a campaign like #saynotochlamydia or #chlamydiaisnotourfuture.”
“Could you handle being grossed out by pictures [of STIs]?” I asked a group of 16 – 18 year old boys. “Yeah, if they see pictures and realise they may have it, they’d wanna get a check up.” There was a suggestion that it could be featured in Shortland Street, depicting ordinary people getting an STI. “You should get someone who’s had chlamydia to talk about it,” said one boy. In this group we then went on to talk about the symptoms you might get if you had an STI, closely followed by the different sizes, shapes, colours and flavours of condoms that a boy called Kevin [pseudonym] had.
As a country we ratified a Treaty called the United Nations Convention on the Rights of the Child (UNCRC) back in 1993. Article 12 of the UNCRC states that children have the right to an opinion in all matters that affect them. As we know that chlamydia predominantly infects children and young people, they should have a say in how they are taught about the issue. My sessions with young people talking about STIs both individually and in groups have always been informative and very helpful from a health professional perspective. I often quote the saying from Phillip McMillan Bowse who was a contributor to England’s Eden project: “If you want to achieve the impossible – give it to the young, because they don’t know it can’t be done.”
About
Dr Karen Hoare is a Nurse Practitioner for children and young people and also a Senior Lecturer at the University of Auckland.
What is InfectedNZ?
Hey, Aotearoa. It’s time we had a chat about infectious diseases and what we’re going to do about the looming antimicrobial armageddon. That’s why we’ve asked leading health, social and economic researchers, and people with personal stories, to help us get real about our vulnerability and discuss solutions. Follow their blogs right here at tepunhahamatatini.ac.nz and watch the conversation spread across social media with #infectedNZ.
Backing it all up, wherever possible, is data from the good folk at Figure.NZ. Their super duper charts are based on data sourced from public repositories, government departments, academics and corporations. Check out their #infectedNZ data board and sign-up to create your very own data board on any topic that floats your boat.

Healthy Homes Project
My name is Nick Pattison and I would like to share with you a citizen science investigation that I was involved in with my previous school Rongomai Primary, called the Healthy Homes Project. The project was created out of conversations between myself and other community members in Otara about the state of local housing in South Auckland, and was magnified at the time by the death of a two-year-old girl in Otara with poor health conditions linked to her damp state home. Something needed to be done.

Dr. Peter Buchanan and students at Landcare Research. Photo taken by Nick Pattison.
I contacted COMET for support and they provided a wonderful project manager, Dr. Sarah Morgan who helped run the project. She contacted local high schools to see if any would be interested in collaborating with us to have students test their homes to investigate the types and amounts of mould. Nicole Stevens from Manurewa High agreed to use her health academy, and at that time there had been no data gathered on South Auckland homes.
The project kicked off with a visit to the local Landcare Research facility, where my students met with researchers to learn how to collect test swab samples and to analyse them for the presence of mould. They then co-designed the method of the investigation. The project included really cool aspects of science – mycology (the study of fungi), modern genetic sequencing, and using microscopes and lab equipment not available in schools. Each student went home after this training and lab induction session and took swab samples from their bedrooms, the living room, the kitchen, and two other rooms of their choice.
The key results from the project
- 18 out of 22 homes were mouldy, and all had the right temperature and humidity to grow mould;
- the mould was readily recovered in a form that was likely to cause harm;
- three different yeasts described as “emerging human pathogens” were found;
- bacteria recovered from some of the homes was resistant to antibiotics; and
- Landcare Research says the homes with the yeasts and the antibiotic-resistant bacteria will need further investigation.
Landcare Research, which helped analyse the samples from the homes of 22 students, did not expect some of the results. Plant pathologist Dr Stanley Bellgard helped the students with their project, Nirvana Healthcare sponsored the project by giving them swabs, and SouthSci funding, which is the South Auckland pilot of the Participatory Science Platform, an initiative under Curious Minds, allowed us to purchase tools called an ibutton to measure temperature and humidity, and produce communication material to share our work.
In the houses, the mould spores were actively in the air and these are the things that we inhale. Mostly it’s not an issue because we are healthy and our wellbeing is intact and these moulds generally don’t have an impact on us. But if we are mentally stressed, physiologically stressed, malnourished or suffering dehydration, or have some predisposition to disease, then these moulds can induce a downward spiral of health.
Fourteen different kinds of mould were found across 18 of the 22 houses the students lived in, but when we looked at some of the yeasts we recovered, they really surprised us because we found three different yeasts that were emerging human pathogens.
Finding yeasts in the study had not been considered but they did have the potential to cause harm. Mr Bellgard said these yeasts, which included Candida parapsilosis and Rhodotorula, were linked to ill-health when combined with other complications, but there was another big surprise to come. We recovered three bacteria that had antibacterial resistance. This was also quite a surprising find beyond the yeast being a threat to health. These antibiotic resistant bacteria can be really, really challenging, especially in a hospital environment.
We deliberately did not look at whether the homes were private or state houses as we’re not trying to stereotype any group within our community and anonymity in scientific research for personal safety is very important. We just want to look at all homes to make sure they are healthy. We wanted the results to be anonymous, so no one could be targeted as having a ‘dirty’ or ‘unhealthy’ home.
The study also revealed the coldest home a student lived in – as measured on a September day at 6am – was 12°C. According to the Asthma Foundation, there is an increased risk of respiratory disease when homes fall below 16°C. The highest humidity recorded was 85 percent.

Rongomai Primary student Junior Wilson. Photo: RNZ / Kim Baker Wilson.
One of my students from Rongomai, Junior Wilson, aged 11, unveiled some of the findings on stage at a Manurewa High School prizegiving. Afterwards he gave interviews for RadioNZ, New Zealand Herald, Stuff, and Manukau Courier amongst others. Since the investigation we were successful in obtaining an Unlocking Curious Minds grant to work with Manurewa High, Landcare Research, and Otago University to investigate the amount of dust mites in homes as this acts as a more reliable model for the health condition of homes.
About
Nick Pattison is the Director of STEM Kauri Flats
What is InfectedNZ?
Hey, Aotearoa. It’s time we had a chat about infectious diseases and what we’re going to do about the looming antimicrobial armageddon. That’s why we’ve asked leading health, social and economic researchers, and people with personal stories, to help us get real about our vulnerability and discuss solutions. Follow their blogs right here at tepunhahamatatini.ac.nz and watch the conversation spread across social media with #infectedNZ.
Backing it all up, wherever possible, is data from the good folk at Figure.NZ. Their super duper charts are based on data sourced from public repositories, government departments, academics and corporations. Check out their #infectedNZ data board and sign-up to create your very own data board on any topic that floats your boat.
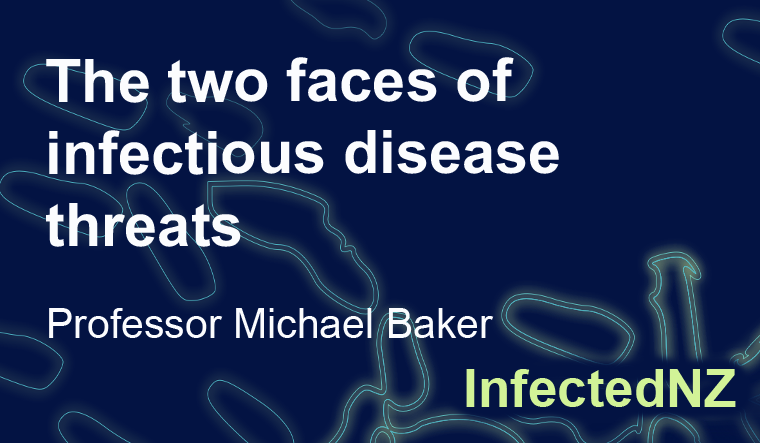
The two faces of infectious disease threats: we need to respond to both
Infectious diseases are far from defeated. They pose a unique health threat because they are caused by living micro-organisms. This biological fact has two important consequences: firstly it means that these micro-organisms are constantly evolving to exploit new ecological opportunities, and secondly they are transmissible (they spread from person-to-person and from infected animals and contaminated environments).
The constant evolution of micro-organisms is one of the drivers of emerging infectious diseases. There are more than 1400 species of infectious organism known to cause disease in humans [1]. New emerging infectious diseases most commonly arise from infections in animals [1]. These organisms are also evolving in other ways, including the development of antimicrobial resistance [2]. The net effect is a constantly changing set of emerging infectious diseases which often need an urgent public health response that has to be adapted to the characteristics of each new threat.
The transmissible nature of infectious diseases also creates many features that distinguish them from non-communicable diseases (NCDs). An infectious disease case can become the exposure for other potential cases, which is not the situation for NCDs such as cancer and heart disease. This transmission risk creates the need for specialised infection control staff in healthcare institutions. On the positive side, infectious diseases can sometimes be eradicated as a human health hazard if transmission can be stopped, as has occurred with smallpox and will hopefully occur with poliomyelitis and other pathogens in the future [3].
The two faces of infectious diseases
The unique features of infectious diseases mean that they manifest two distinct patterns of disease threats.
The first face of infectious diseases is their appearance as a seemingly endless series of new threats that surprise and sometimes terrify us. The most frightening manifestations are pandemics which, by definition, are epidemics that spread to affect multiple geographic regions. These may take the form of new epidemics of known pathogens such as pandemic influenza H1N1, Ebola and Zika, or previously unknown pathogens such as SARS and MERS [4]. Far more common are local epidemics of familiar diseases, which result in several hundred reported outbreaks each year in New Zealand [5].
The second face of infectious diseases is the one that gets less attention, but ultimately accounts for far more human disease and premature death. This is the ongoing impact of established endemic pathogens. Infectious diseases remain the commonest cause of hospitalisation in NZ, accounting for 27% of acute and arranged admissions [6]. The main categories are respiratory, skin and gastrointestinal infections. The highest rates of these infections are in the most vulnerable populations, particularly the young and elderly. They also show a marked social gradient with higher rates among Māori and Pasifika and households living in more deprived neighbourhoods [6].
Responding to infectious disease threats
An effective response to infectious diseases requires us to manage their two faces: the emerging hazards and the ongoing established threats to the health of vulnerable populations.
Our capacity to manage emerging infectious disease threats is difficult to fully access until it is tested, though simulation exercises can help. New Zealand has multiple recognised outbreaks each year reported via its national outbreak recording system. Most are relatively small, well-contained food and water borne outbreaks [5]. An exception was the recent Havelock North campylobacteriosis outbreak which was remarkable in terms of its size (estimated at 5,200 cases). This event reminded us of our vulnerability to drinking water contamination and the need to consider increasing pressures on our environment, notably the impact of climate change and intensification of animal-based agriculture [7]. The Havelock North outbreak comes in the context of a much larger, prolonged epidemic increase in campylobacteriosis caused by rising production and consumptions of contaminated fresh poultry meat [8].
A major advance in managing globally important emerging infectious diseases has come from the International Health Regulations 2005. This agreement requires all WHO member states to assess and report emerging infectious diseases that have potential to develop into public health emergencies of international concern (known as PHEIC) [9]. Since the regulations came into force there have been four such emergencies: pandemic influenza H1N1 in 2009, polio in 2014, Ebola in 2014, and Zika in 2016. Of these, only pandemic influenza spread to NZ, though its impact was comparable to normal seasonal influenza [10].
The Ebola pandemic in West Africa was a grim reminder of the need for a more proactive approach to managing emerging infectious diseases. There is now growing support for the Global Health Security Agenda which is placing more emphasis on prevention and ensuring adequate health services for people living in low and middle-income countries [11].
Given the dynamic nature of emerging infectious diseases, New Zealand needs to constantly review and refine its response capacity. We have not been good at documenting lessons from major epidemics and pandemics in the past [12]. The Independent Inquiry into the Havelock North campylobacteriosis outbreak is therefore a welcome opportunity to identify and act on the lessons learned from such events [7].
In terms of the second face of infectious diseases, there is considerable evidence that New Zealand has performed poorly in recent times. We have a history of increasing rates of hospitalisation for serious infectious diseases, particularly during the 1990s. We also have very high levels of inequality in rates of infectious diseases generally, particularly across ethnic and socioeconomic groups [6]. The most extreme example is rheumatic fever where Māori and Pasifika are 14 to 21 times more likely to develop the disease compared to other New Zealanders [13].
Addressing New Zealand’s high rates of serious infectious diseases of poverty is a major challenge. This situation requires a highly strategic approach with engagement from multiple Government agencies. An effective response includes achieving high coverage with vaccines and other interventions targeting specific infections like pneumococcal disease and rheumatic fever. Ultimately, the biggest gains depend on improving major health determinants, such as housing [14] and access to healthcare, and reducing child poverty [15].
Fundamental to all of these measures is having the infrastructure to develop and sustain the policies and programmes needed to manage both the epidemic and established faces of infectious diseases. This infrastructure requires a high level of multi-sectoral coordination, effective multidisciplinary networks including One Health [16], and good linkages to international prevention and control initiatives. This capacity needs to be supported by highly developed surveillance systems, a skilled workforce (including infectious disease specialists, microbiologists and veterinarians), and a vigorous programme of operational research to keep ahead of these infectious disease threats. Increasing levels of antimicrobial resistance will provide an important test of New Zealand’s ability to mount an effective response to a serious emerging infectious disease problem [17].
Conclusion
Infectious diseases are a unique threat to human health and wellbeing. We know a lot about how to manage this challenge but are not necessarily acting on this knowledge. Important gaps include the need to address diseases of poverty and the need to keep investing in surveillance and coordinated response capacity that can detect and manage emerging diseases and pandemics.
About
Michael Baker is a public health medicine specialist and professor of public health at the University of Otago, Wellington. He is Director of the University’s Health Environment Infection Research Unit (HEIRU), Co-director of He Kainga Oranga / Housing and Health Research Programme, and Chief Investigator at the Australian Centre for Research Excellence on Integrated Systems for Epidemic Response (ISER).
What is InfectedNZ?
Hey, Aotearoa. It’s time we had a chat about infectious diseases and what we’re going to do about the looming antimicrobial armageddon. That’s why we’ve asked leading health, social and economic researchers, and people with personal stories, to help us get real about our vulnerability and discuss solutions. Follow their blogs right here at tepunhahamatatini.ac.nz and watch the conversation spread across social media with #infectedNZ.
Backing it all up, wherever possible, is data from the good folk at Figure.NZ. Their super duper charts are based on data sourced from public repositories, government departments, academics and corporations. Check out their #infectedNZ data board and sign-up to create your very own data board on any topic that floats your boat.
References
- Taylor LH, Latham SM, Woolhouse ME: Risk factors for human disease emergence. Philosophical transactions of the Royal Society of London Series B, Biological sciences 2001, 356(1411):983-989.
- Williamson DA, Heffernan H: The changing landscape of antimicrobial resistance in New Zealand. N Z Med J 2014, 127(1403):41-54.
- Centers for Disease C, Prevention: Global Disease Elimination and Eradication as Public Health Strategies. Proceedings of a conference. Atlanta, Georgia, USA. 23-25 February 1998. MMWR supplements 1999, 48:1-208.
- de Wit E, van Doremalen N, Falzarano D, Munster VJ: SARS and MERS: recent insights into emerging coronaviruses. Nature reviews Microbiology 2016, 14(8):523-534.
- The Institute of Environmental Science and Research Ltd: Notifiable Diseases in New Zealand: Annual Report 2014. In. Porirua, New Zealand The Institute of Environmental Science and Research Ltd.; 2015.
- Baker MG, Telfar Barnard L, Kvalsvig A, Verrall A, Zhang J, Keall M, Wilson N, Wall T, Howden-Chapman P: Increasing incidence of serious infectious diseases and inequalities in New Zealand: a national epidemiological study. Lancet 2012, 379(9821):1112-1119.
- Woodward A, Hales S: Note to the Havelock North Inquiry – Think Big. In: Public Health Expert Blog, https://blogsotagoacnz/pubhealthexpert/2016/09/26/note-to-the-havelock-north-inquiry-think-big/. 2016.
- Baker M, Wilson N: NZ’s long-running Campylobacter epidemic from poultry: Now with antibiotic resistance. In: Public Health Expert Blog, https://blogsotagoacnz/pubhealthexpert/2015/12/19/nzs-long-running-campylobacter-epidemic-from-poultry-now-with-antibiotic-resistance/. 2015.
- Baker MG, Fidler DP: Global public health surveillance under new international health regulations. Emerg Infect Dis 2006, 12(7):1058-1065.
- Baker MG, Wilson N, Huang QS, Paine S, Lopez L, Bandaranayake D, Tobias M, Mason K, Mackereth GF, Jacobs M et al: Pandemic influenza A(H1N1)v in New Zealand: the experience from April to August 2009. Euro Surveill 2009, 14(34):pii=19319.
- Heymann DL, Chen L, Takemi K, Fidler DP, Tappero JW, Thomas MJ, Kenyon TA, Frieden TR, Yach D, Nishtar S et al: Global health security: the wider lessons from the west African Ebola virus disease epidemic. Lancet 2015, 385(9980):1884-1901.
- Wilson N, Summers JA, Baker MG: The 2009 influenza pandemic: a review of the strengths and weaknesses of the health sector response in New Zealand. N Z Med J 2012, 125(1365):54-66.
- Gurney JK, Stanley J, Baker MG, Wilson NJ, Sarfati D: Estimating the risk of acute rheumatic fever in New Zealand by age, ethnicity and deprivation. Epidemiol Infect 2016:1-10.
- Howden-Chapman P: Home Truths: Confronting New Zealand’s Housing Crisis. Wellington: Bridget Williams Books; 2015.
- Boston J, Chapple S: Child poverty in New Zealand. Wellington: Bridget Williams Books; 2014.
- Gibbs EP: The evolution of One Health: a decade of progress and challenges for the future. The Veterinary record 2014, 174(4):85-91.
- Pullon HW, Gommans J, Thomas MG, Metcalf S, Grainger R, Wild H: Antimicrobial resistance in New Zealand: the evidence and a call for action. N Z Med J 2016, 129(1444):103-110.

Antibiotic resistance is looming but we can protect the children
In another life I used to sit on teams that developed something called ‘clinical guidance’. We would draw together published evidence and make recommendations on what best practice should look like. I sat on one such group for rheumatic fever.
Rheumatic fever (RF) is a disease now seen mainly in developing countries (apart, of course, from the poor pockets of New Zealand where it runs at near epidemic rates). If rheumatic fever is not caught early, it leads to rheumatic heart disease (RHD) that in turn leads to serious heart damage – the sort of damage that can see people die in their late 30s.
On this rheumatic fever clinical guidance group that I sat on, I heard a story about the antibiotics that are used to treat the disease. I heard that many of the kids with rheumatic fever live rurally and getting treatment to them is a challenge. I heard that the antibiotics needed to be kept at temperatures lower than a few degrees. I heard that these antibiotics are often driven around the dusty roads of rural New Zealand in the height of a Kiwi summer, eventually getting to families warm and possibly no longer effective. What I heard was that kids who needed the antibiotics the most were being let down by basic logistical stuff-ups.
Is this story representative? Are vulnerable low-income children missing out on antibiotics? And if children are already missing out on antibiotics when they need them in New Zealand, how will population-wide antibiotic resistance affect these children? Children who, coming from low income families, are already at greater risk of infectious disease than other children in New Zealand?
The story for low-income kids and antibiotics is complex & resistance is not going to make things better
Children living on low incomes in New Zealand have more infections (requiring antibiotic treatment) than other children. Rheumatic fever is one disease they contract at higher rates, especially as overcrowding and poor quality housing continues unabated in Auckland. But we also know from the Child and Youth Epidemiology Service, who reports on child health in New Zealand each year, that skin infections plague children from poorer communities. We also know that respiratory infections put them in hospital at a much greater rate than others. And when diseases like measles break out, they tend to do so in low-income communities, with the bulk of the health effects being felt by the children of low -income families. These are the children who need antibiotics and need them to work. Even with antibiotics, children are still dying in New Zealand from diseases of the third world.
Poor children need antibiotics more than other children, but are they getting them?
The situation is complex. We have data showing us that antibiotic prescribing in Counties Manukau in Auckland (a large low-income community) is greatest for those living in overcrowded households [1]. Overcrowding is one of the indicators of poverty in New Zealand.
Below we can see the relationship between antibiotic prescribing and overcrowded households in Counties Manukau. We see as the ratio goes up (representing more people in a bedroom) antibiotic scripts rise too. This finding is understandable in the context of how infectious diseases spread – through close contact with other infected people.
But does the higher prescribing rate of antibiotics for the vulnerable mean all the children who need them are also getting them? A prescription for antibiotics is not a measure of antibiotics used. We can look at the dispensing of antibiotic use for some clues.
Researchers examined the dispensing of antibiotics (pharmacies filling a prescription) in another part of New Zealand. The town was a rural town at least an hour from other towns with a pharmacy. Researchers found that individuals living in lower income areas of that community were much less likely to be dispensed antibiotics.[2]
So we see that infections are higher for low-income children in New Zealand, and prescriptions may also be higher, but that actual use may be lower for this group.
Low-income children do have the worst access to healthcare in New Zealand
The data present a complex picture, but not one those working in health are unfamiliar with. It highlights what we see across all societies – those that need healthcare the most may be accessing it the least. We call it the inverse care law.
We can speculate based on this evidence what is happening with low-income children in New Zealand. It may be that children of low-income families are getting to the GP but not getting the antibiotics on board. It may be that even getting to the GP is such a challenge that low-income children are not being seen till very late – we certainly know that low-income children see medical professionals a lot later down the path of an infectious disease making treatment more difficult and spread and recurrence more likely.
It may be that low-income families cannot easily access the antibiotics even once they are prescribed. We know that in New Zealand the children who don’t get to the GP because they have no transport (a measure of material deprivation & poverty) are the children of low-income parents, as the data below demonstrates.
New Zealand has a government funded primary healthcare system, with free GP visits for children under 13 and low cost prescriptions. But in reality, there is an imbalance in who accesses this funded healthcare. It not just healthcare itself that costs families – the opportunities to access the care also costs: time off work; access to transport; being free of other health debts and social stigma; having someone who can care for any other children. These are all resources a parent needs to be able to draw upon to access healthcare for their child. In many cases, low-income families have fewer of all of these resources. While the data has gaps, it certainly indicates that there is likely to be a problem in terms of low-income children’s access to and use of antibiotics when they need them.
What happens when we add antibiotic resistance into the mix?
Nothing good. For children who need antibiotics the most, resistance has the most serious impact. For a group of children who are already disadvantaged by being poor, we have at least always had the hope that, if they can equitably access timely treatment, we can overcome some of the disadvantage they suffer. With resistance looming this will not be the case, and the children who will die at the greatest rate from what are now just everyday infections will of course be those already getting the worst deal from the imbalance in New Zealand society. But we can do something about this.
What can be done?
As always the ambulance parked at the bottom of the cliff will not assist us here. Research into antibiotic alternatives needs funding, and a huge reduction in unnecessary antibiotic use is needed (including in animals and farming).
However, there is something we can do right now for children living on low-incomes – we can correct the imbalance in their health outcomes through strong policy. Research tells us there are many effective ways to address this imbalance, and most of them lie outside of the control of the health workforce.
Bringing families with children up over the various poverty lines using unconditional cash assistance is an area that has significant potential to improve children’s health. Housing affordability, quality and access is another area with serious potential to improve low-income children’s health. The best evidence shows that improvements to the physical aspects of the home (e.g., ensuring it is the appropriate size for a family, it can affordably be kept warm, it is insulated and not damp) improves health (especially in those with existing respiratory illness), may improve and promote relationships in the family, and may reduce absences from school or work. The findings are especially applicable in low-income households where the need is greatest.[3]
The end of antibiotics as we know is upon us. However, in the time between their discovery and their demise, let us finally deliver for low-income children a good society, one that ensures we are all already in the same boat as we work to find solutions together.
References
[1] Walls, G., Vandal, A. C., du Plessis, T., Playle, V., & Holland, D. J. (2015). Socioeconomic factors correlating with community antimicrobial prescribing. NZ Med J, 128(1417), 16-23.
[2] Norris, Pauline, Gordon Becket, and Denise Ecke. “Demographic variation in the use of antibiotics in a New Zealand town.” The New Zealand Medical Journal (Online) 118.1211 (2005).
[3] Thomson H, Thomas S, Sellstrom E, Petticrew M. Housing improvements for health and associated socio-economic outcomes. Cochrane Database of Systematic Reviews 2013, Issue 2. Art. No.: CD008657. DOI: 10.1002/14651858.CD008657.pub2.
About
Dr Jess Berentson-Shaw works at the Morgan Foundation Policy Think Tank and writes about science, children and families at The Spinoff Parents. You can follow her on Twitter @DrJessBerentson
What is InfectedNZ?
Hey, Aotearoa. It’s time we had a chat about infectious diseases and what we’re going to do about the looming antimicrobial armageddon. That’s why we’ve asked leading health, social and economic researchers, and people with personal stories, to help us get real about our vulnerability and discuss solutions. Follow their blogs right here at tepunhahamatatini.ac.nz and watch the conversation spread across social media with #infectedNZ.
Backing it all up, wherever possible, is data from the good folk at Figure.NZ. Their super duper charts are based on data sourced from public repositories, government departments, academics and corporations. Check out their #infectedNZ data board and sign-up to create your very own data board on any topic that floats your boat.

Living with MRSA
Wash your hands all you want. Don’t touch things. Clean your cuts and grazes. Be careful all you can… but it won’t make any difference. An open wound will grow; it will cause rashes on your body and your skin to blister and weep. Once it’s in your blood stream, it goes wild, living in there like an uninvited guest crashing your dinner party.
It smells. I can smell it a mile away. Doctors laugh at you, but it definitely has its own smell. It stinks and to get rid of it, to try and get it to leave you completely, is like being entrenched in a war where no matter how hard you try the other side is always tougher.
I spit the words when I say them as it has haunted my life and my family’s life for so long. It enveloped my every waking moment, and every sleepless night, as I wished moment after moment it had never come into our lives.
It’s MRSA, a form of staph bug – a superbug which has nothing I deem “super” about it. To me, super is like a “super hero”, but the only hero when it comes to MRSA is those who battle it or those who are trying to cure it.
My little girl lived in hospital a long time battling gastrointestinal failure and the complications that accompany it. We were definitely no strangers to infections, spending weeks on end on all order of antibiotics for every different thing over years and years. That was my biggest worry then, and now. People used to say MRSA, MSBL, C.diff, and I used to just switch off; my child didn’t have them, so it didn’t matter.
Then one day, while she battled a burst gall bladder and just wouldn’t show signs of improvements as she had infection after infection, a doctor came into the room, gowned, gloved, and masked and said she has MRSA in her blood stream and it’s growing in her line and tubes.
Oh shit, I said. That’s all I said. I still didn’t register how much it would affect our lives.
We lived in the hospital and were always around and about, playing with nurses, in the play room; anything to pass the time. Now, once she recovered from the gall bladder incident, we couldn’t play; she still grew MRSA. Too many lines and tubes. No more playing with other children in hospital. No more playroom. We were stuck – isolated with a bug that was growing more resistant by the day and scared of spreading it around. And, of course, following strict hospital protocol around such bugs.
But, reality was, that’s nothing compared to the impact it had on my child physically. No longer are you just battling a medical condition but a serious infection on top. It rules your life and all the choices you make.
It leaks and oozes. Then you change a dressing and after multiple changes it then gets raw and oozy. Years of constant infections followed, and traumatic moments that I won’t relive here.
You think with an infection that doctors just get an antibiotic and treat it. If that doesn’t work, they will have another. The thing about a bug like this is you can get to the end of antibiotics, and those IVs take longer and longer before a solution is found. Within a week, after more and more attempts, it then doesn’t work at all. The bug lays dormant then sneaks up and flares up when you least expect it. One day nothing. Then you look the next day and see the familiar pale yellow ooze leaking out from a line and your heart sinks. You know it will get worse before it gets better.
The other thing people don’t get is the toll endless antibiotics takes on a body: thrush, reactions, tummy pain, puffiness, blowing lines. Endless and yet unavoidable. The constant fear of what next eats away at you as well as the constant worry about treatments not working. Even short term IV lines grew MRSA as we lost line after line and our vein access went from minimal to pretty much nothing.
The thing is, it’s a bug, you catch it. That brings a whole different sort of grief and anger compared to a condition you are born with or develop. Anger ruled my life. When MRSA flared up, I was often so scared as the violent convulsions from temperatures would take hold from another infection in the line. It had to be someone’s fault. I found people to blame and, to an element, I still do. But it’s nothing compared to the guilt and blame you place on yourself. As a mother, I should protect my children – the most precious things in my life.
Yet, while in my care, my child contracted a bug that was ravishing her poor little body. Did I not wash my hands enough? Did I not watch procedures close enough? Should she have sat on the ground when she did? So many stupid questions. When I’m not exhausted, and as life moves on, I know it’s a bug that’s everywhere. The longer you are in hospital, the more immune-suppressed you are, or just pot luck, you’re likely to contract a superbug. I just wish it hadn’t grown on my child. I wish it didn’t exist at all.
We don’t have a line now. The last growth of MRSA was followed by thrush in her blood stream and it meant the final access was to be removed. Reality is, any line is going to be an area likely to grow this bug.
The not sleeping at night as you check continuously for a line infection; the constant changing of painful dressing; the fear that this could be in her blood (and not picked up earlier) all dulled, as the risk is so much lower. But, unfortunately, it still rears its head, but in more fiddly ways. A skinned knee ends up more manky, the longed-for pierced ears mucky and taking longer to heal. It’s a relief to sleep easier at night but also a battle to keep a child with gut failure line-free.
Something good I realised, just the other day, was how improved her quality of life has become now that she hasn’t got a line oozing MRSA continuously. That bug took such a toll on her body that she was bloated and puffy from constant meds.
It’s been a really big and cruel lesson to learn how important the research being done to treat and cure Staph bugs is. It’s something you just wish no one had to deal with. It’s something I wish my family never had to deal with.
We need to find a cure.
About
This post was provided by parent Tiff Mora.
What is InfectedNZ?
Hey, Aotearoa. It’s time we had a chat about infectious diseases and what we’re going to do about the looming antimicrobial armageddon. That’s why we’ve asked leading health, social and economic researchers, and people with personal stories, to help us get real about our vulnerability and discuss solutions. Follow their blogs right here at tepunhahamatatini.ac.nz and watch the conversation spread across social media with #infectedNZ.
Backing it all up, wherever possible, is data from the good folk at Figure.NZ. Their super duper charts are based on data sourced from public repositories, government departments, academics and corporations. Check out their #infectedNZ data board and sign-up to create your very own data board on any topic that floats your boat.
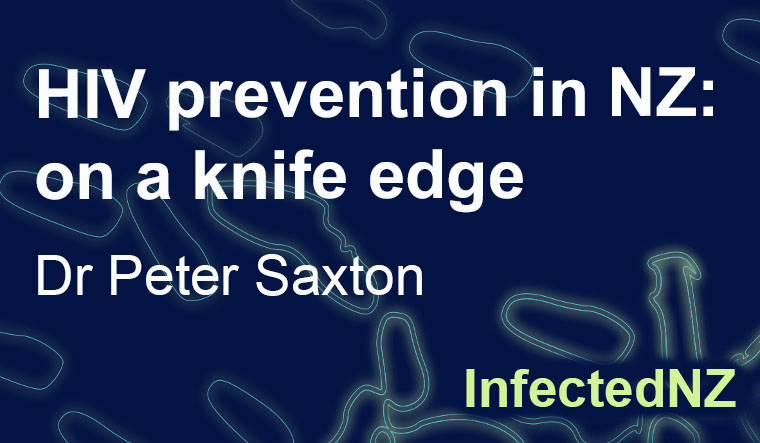
HIV prevention in NZ: on a knife edge
HIV seems like a scourge of the eighties, but today new HIV diagnoses and the costs of medication are escalating out of control in New Zealand. The world’s most dangerous infectious disease epidemic of the modern era is being revived, fuelled by invisibility, indifference and inaction.
HIV has a terrifying ability to evolve and evade control when our guard is down. The virus has killed 40 million people worldwide. 2.5 million people contracted HIV in 2015. Three decades later we still have no vaccine and no cure.
The fact is HIV is exceptionally well adapted to spread between humans. The virus is transmitted through sexual behaviour and injecting drugs, behaviours that are not easy to change. Infection is often asymptomatic, so people need to be tested to confirm if they have the virus. HIV is most infectious shortly after someone becomes infected, when they are probably unaware that they have it. Finally, HIV is subject to cultural taboos, triggering embarrassment and discouraging open conversations.
So despite three decades of scientific knowledge we’re again witnessing expanding epidemics across the globe; from mature epidemics in the UK to new epidemics like the Philippines, where transmission since 2005 has been explosive.
The latest trends here are alarming. Last year New Zealand recorded the highest number of annual HIV diagnoses ever, the fourth increase in a row. In gay and bisexual men, who account for 80% of our epidemic, annual HIV cases contracted locally are now four times higher than at the epidemic low point in 2000.
Treatment expenditure is also soaring, doubling in the last 6 years from $16.8 million in 2011 to $32.8 million in 2016. It’s estimated that one person diagnosed with HIV at age 20 could cost Pharmac over $800,000 in antiretroviral treatments over their lifetime. It’s why HIV prevention makes great fiscal sense: for every infection averted we’d be able to fund other much needed medication for New Zealanders.
It’s disappointing because we’ve got a proud record that risks being squandered. When HIV first made it to New Zealand over thirty years ago, the country responded boldly. Interventions were based on evidence and we agreed on rational policies, bi-partisan political engagement and urgency. Moralism was rejected in favour of science and cooperation.
The result was an international infection control success story: New Zealand was one of the first countries in the world to report a decline in AIDS. We had among the lowest rates of HIV diagnoses in gay and bisexual men internationally, and we still have the lowest rate of HIV among people who inject drugs.
HIV’s comeback is driven by a roll-call of big issues confronting contemporary societies.
Internet dating’s hyper-connectivity has been exploited by HIV and other sexually transmitted infections, spreading through sexual networks in clusters and to individuals previously distant from transmission epicentres.
Social media could be used to empower HIV prevention but Google, Facebook and Apple have blocked condom and HIV testing advertisements due to their “offensive content”. That means content has to be clinical and sanitised, reducing its relevance to target audiences.
Pornography is increasingly accessible and unregulated, but tends to promote condomless sex.
Pharmaceuticals have thankfully transformed life expectancy to near-normal with early diagnosis. But they also make HIV seem less visible and less threatening, and their expense ties up scarce health resources, leaving less money for safe sex programmes and research.
Stigma compounds that invisibility, silencing the voice of people living with HIV and deterring those at risk from seeking testing and prevention resources.
And rising consumption of recreational drugs, particularly methamphetamine, can facilitate unprotected sex and increases HIV transmission risks if unsterilised injecting equipment is shared.
The upshot is that nowadays HIV prevention operates in a very challenging environment. The accumulation of these small shifts and unintended consequences has allowed HIV to persist and reassert itself.
Likewise we shouldn’t forget why HIV is described as “the most political of diseases”. Controlling HIV means confronting established societal power structures head on, be they gender (men controlling women’s sexual norms), age (adults limiting young people’s access to sex education) or sexuality (gay communities denied sufficient resources because of heterosexism and homophobia).
In New Zealand, the greatest disparities in HIV are found among sexual orientation minorities. Our country has a favourable history promoting equal rights for gay communities so it’s unacceptable that the health status of the same groups doesn’t appear to enjoy the same commitment. If studies suggested that 1 in 15 heterosexual participants had HIV, with 1 in 5 of these undiagnosed, I’m left wondering if there’d be more urgency to respond?
What’s exciting and frustrating HIV experts here is that New Zealand could turn this around quickly. Scientific developments in the last two years mean that it is possible to virtually eliminate HIV transmission in New Zealand within 15 years. These developments centre on continued safe sex promotion, more frequent HIV testing, and adding two new tools to the mix.
The first tool is immediate antiretroviral treatment for people who test HIV positive. That’s because recent studies show that immediate rather than delayed treatment considerably improves long term health, and also suppresses the amount of virus in the body (called the HIV viral load) to the point where a person is almost uninfectious. In the “PARTNER” prospective cohort study, no linked transmissions were found from the HIV positive participants who had fully suppressed virus to their uninfected partner, even after 58,000 sex acts that didn’t involve condoms.
The second tool is offering the same HIV treatments as daily pre-exposure prophylaxis (PrEP) to the small number of uninfected individuals at very high risk of contracting HIV. Several studies have shown that PrEP reduces the risk of acquiring HIV by 86-92%, benefitting these individuals and also preventing chains of transmission to others: a population effect.
The World Health Organisation recently recommended immediate treatment on diagnosis, and countries such as the United States, France and Norway offer funded access to PrEP. In contrast, New Zealand government agencies have been slower to act, not yet approving immediate treatment or endorsing PrEP.
We urgently need re-engagement and action on HIV. We need to evolve and modernise our responses just as HIV has evolved from a frightening disease causing panic and fear, to a silent epidemic flying under the radar but harming our communities and health budgets.
At a time when we finally have the tools necessary to eradicate transmission, we literally can’t afford complacency to undo all the good work of our predecessors.
About
Peter Saxton is a Senior Research Fellow with an interest in HIV prevention and sexual health. He has a PhD in public health and is the inaugural New Zealand AIDS Foundation Fellow at the University of Auckland.
What is InfectedNZ?
Hey, Aotearoa. It’s time we had a chat about infectious diseases and what we’re going to do about the looming antimicrobial armageddon. That’s why we’ve asked leading health, social and economic researchers, and people with personal stories, to help us get real about our vulnerability and discuss solutions. Follow their blogs right here at tepunhahamatatini.ac.nz and watch the conversation spread across social media with #infectedNZ.
Backing it all up, wherever possible, is data from the good folk at Figure.NZ. Their super duper charts are based on data sourced from public repositories, government departments, academics and corporations. Check out their #infectedNZ data board and sign-up to create your very own data board on any topic that floats your boat.
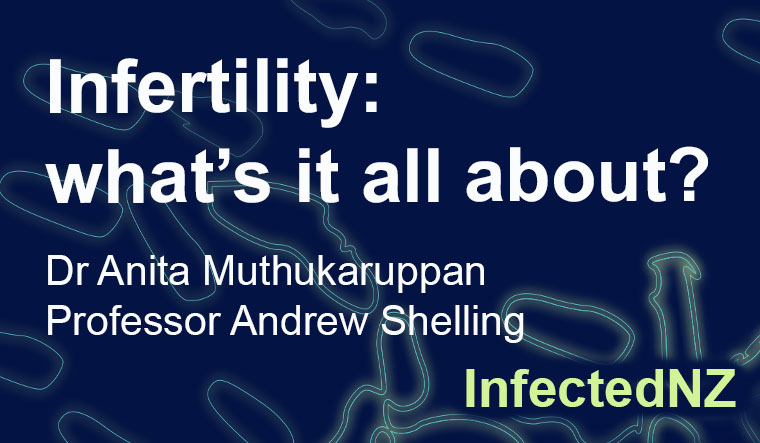
Infertility: what’s it all about?
Infertility is defined as the inability for a couple having regular unprotected sexual intercourse to have a baby. Globally, the rate of fertility is declining but infertility still affects one in seven couples in New Zealand. In general, humans are generally not considered to be an especially fertile species, with a potential capability of 20-30% reproductive success with each menstrual cycle, albeit in a small window of opportunity for fertilisation of 5-6 days within each menstrual cycle. Fertility is highest in women in their 20s, and the rate of fertility declines with age, declining even more rapidly in a woman’s 40s, until the age of 50 where menopause occurs. This is when all of a woman’s eggs are exhausted within the ovaries and reproductive capacity ceases.
Women are increasingly delaying childbearing usually due to social and economic reasons. This, coupled with a gradual decline in fertility, means that other causes of infertility can become a significant issue, including having lifestyle factors such as obesity and smoking and sexually transmitted infections such as chlamydia and gonorrhea. These infections are further complicated by the fact that carriers of these infections can remain symptomless.
Chlamydia: the tip of the infertility iceberg
Chlamydia is the most common sexually transmitted infection in New Zealand, caused by the bacterium Chlamydia trachomatis. Chlamydia can be transmitted during vaginal, anal, or oral sex, and can be passed from an infected mother to her baby during childbirth. Chlamydia infections do not exhibit any symptoms in approximately 70% of women and 25% of men. While a chlamydia infection can be effectively cured with antibiotics, if left untreated there can be serious long-term reproductive consequences.
Approximately half of the women with a symptomless chlamydia infection will develop pelvic inflammatory disease, which is a generic term for infection of the uterus, fallopian tubes, ovaries and its surrounding tissues. Pelvic inflammatory disease can result in scarring and permanent damage of the reproductive organs, which can cause serious complications such as chronic pelvic pain, ectopic pregnancies (when an embryo implants in other parts of the female reproductive tract apart from the uterus), and infertility, especially with repeat infections. In men, if left untreated, chlamydia can cause pain, swelling and inflammation in the testes and urethra, and also lead to infertility.
According to a recent report to the Ministry of Health, laboratory surveillance efforts by the Institute of Environmental Science and Research Limited (ESR) showed that chlamydia was the most commonly reported sexually transmitted infection in 2014. With a national rate of 629 cases per 100,000 population, our rates are double those of Australia and the UK. 83% of cases reported were aged between 15 and 29 years. Chlamydia rates for males increased by 24.9% in the 40 years and over age group, and increased by 10% in the 25–29 years age group, but were generally stable in all other age groups. Chlamydia rates decreased in all age groups for females. However, there was more than twice the number of cases of chlamydia in women than in men. The national rate for females was 869 per 100,000 population (19,986 cases) compared to males that was 375 per 100,000 population (8275 cases).
Gonorrhoea: not quite the round of applause you’d like…
Gonorrhoea (commonly known as the clap) is a sexually transmitted infection caused by the bacterium Neisseria gonorrhoeae, that can be transmitted during vaginal, anal, and oral sex, and from an infected mother to her baby during childbirth. The usual symptoms in men are a burning sensation when urinating and penile discharge. Women, on the other hand, may have vaginal discharge and pelvic pain. Gonorrhoea infections do not exhibit any symptoms in approximately 50% of women and up to 5% of men. If left untreated, the infection can spread locally, causing inflammation in the testes in men, or pelvic inflammatory disease in women. Gonorrhoea can also spread to affect joints and heart valves in the body.
According to the ESR survey, in 2014 our national gonorrhoea rate was 70 cases per 100,000 population, with 73% of those cases being in people aged between 15 and 29 years. The national rate for males was 77 per 100,000 population (1633 cases) and was higher than the national rate for females, that was 62 per 100,000 population (1367 cases). Between 2010 to 2014, there have been increasing rates of gonorrhea in both men and women, in a number of age groups; 40 years and over, and 25–29 years in men, and 15– 19 years and 30–34 years, in women. In this same time period, the numbers of gonorrhoea cases reported in women were highest in the 15–19 years and 20–24 years age groups, and in men were highest in the 25–29 years age group. More concerning is that antibiotic-resistant strains of N. gonorrhoeae have been identified in gonorrhea patients in New Zealand.
Reproductive health is an easily forgotten and underrated area of personal health care. Early recognition and effective treatment of sexually transmitted infections is an important protection against later problems with declining human fertility.
References:
References
- Gimenes F, Souza, RP, Bent, JC et al. Male infertility: a public health issue caused by sexually transmitted pathogens (2015). Nat Rev Urol. 11, 672-687.
- Menon S, Timms P, Allan JA, et al. Human and Pathogen Factors Associated with Chlamydia trachomatis-Related Infertility in Women (2015). Clin Microbiol Rev. 28(4):969-85.
- Newman L, Rowley J, Vander Hoorn S, et al. Global Estimates of the Prevalence and Incidence of Four Curable Sexually Transmitted Infections in 2012 Based on Systematic Review and Global Reporting (2015). PLoS One. 10(12): e0143304.
- Sexually transmitted infections in New Zealand 2014. Institute of Environmental Science and Research Limited.
- Skerlev M, Čulav-Košćak I (2014). Gonorrhea: New challenges. Clin Dermatol. 32, 275-281.
- Markle W, Conti T, Kad M (2013). Sexually transmitted diseases. Prim Care. 40(3):557-87.
- Garnett G, (2008). How much infertility does chlamydia cause? Sex Transm Infect. 84, 157-158.
About
Dr Anita Muthukaruppan is a breast cancer researcher with the Department of Obstetrics and Gynaecology at the University of Auckland. She is a science geek with interests in gynaecological cancers, infertility, pregnancy, sexually transmitted infections, antibiotics, vaccines, carnivorous plants and quantum physics. Shea also loves Sir David Attenborough, Professor Stephen Hawking and Dr Emmett Brown.
Professor Andrew Shelling is Associate Dean (Research) at the Faculty of Medical and Health Sciences, and also head of the Medical Genetics Research Group in the Department of Obstetrics and Gynaecology. His research is primarily interested in understanding the molecular changes that occur during the development of genetic disorders, focusing on breast and ovarian cancer, and reproductive disorders. Andrew enjoys trying to keep up with advances in science.
What is InfectedNZ?
Hey, Aotearoa. It’s time we had a chat about infectious diseases and what we’re going to do about the looming antimicrobial armageddon. That’s why we’ve asked leading health, social and economic researchers, and people with personal stories, to help us get real about our vulnerability and discuss solutions. Follow their blogs right here at tepunhahamatatini.ac.nz and watch the conversation spread across social media with #infectedNZ.
Backing it all up, wherever possible, is data from the good folk at Figure.NZ. Their super duper charts are based on data sourced from public repositories, government departments, academics and corporations. Check out their #infectedNZ data board and sign-up to create your very own data board on any topic that floats your boat.
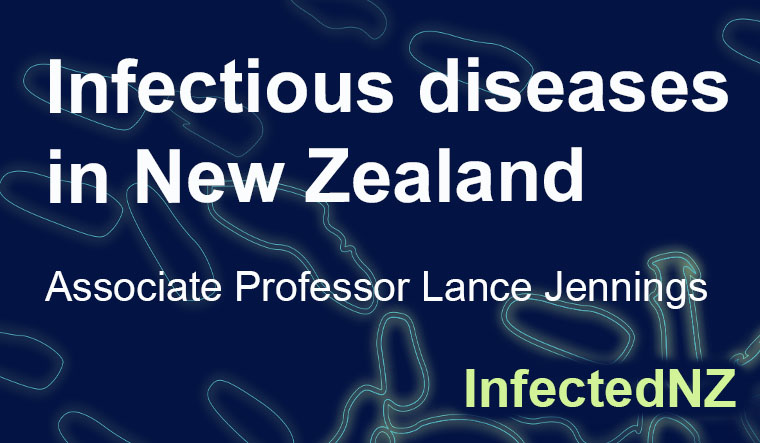
Infectious diseases in New Zealand
In the context of World Antibiotic Awareness week last week (November 14-20) and efforts to raise the awareness of infectious diseases in New Zealand, it is useful to include a discussion on viruses.
Although few treatments and vaccines are currently available for their control, the burden of disease in terms of personal affliction, loss of work or school days, hospitalisation and death is substantial. The viruses that cause human respiratory infections provide a window into the wider issues that we are dealing with regarding infectious diseases in New Zealand at present.
There are over 150 known respiratory viruses which by and large cause mild respiratory symptoms, the most common of which are the Rhinoviruses which infect the upper respiratory tract causing the common cold. Respiratory Syncytial Virus (RSV) is an important cause of more severe lower respiratory tract infections in young children, such as bronchiolitis and pneumonia, resulting in increased numbers of hospital admissions during the winter and spring months when this virus circulates in New Zealand annually. Fortunately a vaccine will be available in the near future for this virus.
Influenza is another respiratory virus which affects New Zealander’s annually. Four viruses are currently circulating: Influenza A(H3N2), A(H1N1)pdm09, Influenza B/Yamagatta and B/Victoria. Typical winter outbreaks and epidemics result in substantial illness, visits to general practitioners, school and workplace absenteeism and hospitalisation of especially young children and the elderly.
Effective interventions against influenza are available, with annual vaccination the most effective for preventing influenza infection. Vaccination needs to be annual as these viruses continue to evolve, undergoing antigenic drift, requiring frequent vaccine composition review and virus composition updating. Vaccines cannot be used in children under 6 months of age, and the best way to protect them is through vaccination of the mother before child birth. The elderly are also an issue as their immune systems are less active and they respond less vigorously to the vaccine. Regardless, vaccination is the most effective way we currently have to protect individuals against influenza.
Antivirals are effective for the treatment of influenza infections, especially if given early after the onset of symptoms. The Neuraminidase inhibitors (Tamiflu and Relenza) are the most common antivirals available. Unlike the use of antibiotics against bacterial infections, where unnecessary use contributes to the development of resistance, misuse of antivirals in general does not drive the development of antiviral resistance and other genetic mechanisms are involved when influenza viruses have developed resistance in the past.
In recent years novel respiratory viruses have caused global concern and have resulted in countries, including New Zealand establishing pandemic preparedness plans. The most recent influenza pandemic was due to the A(H1N1)pdm09 virus which emerged from pigs in Mexico in 2009. Characteristically influenza pandemics have been associated with high mortality (3% of the world’s population died during the Spanish 1918-19 pandemic) and substantial illness. However the 2009 virus was related to a virus that had circulated prior to 1957 and caused generally milder infections. Regardless, the circulation of this new virus did place substantial pressure on our health system over 2009 and the winter of 2010.
The emergence of the avian influenza A(H5N1) virus in Hong Kong in 1997, then re-emergence and spread amongst domestic poultry from late 2003 in many parts of the world remains a concern. Although human infections are rare, they are consistently associated with a 50% mortality rate. Should this virus develop the ability to be transmitted between humans, the outcome would be devastating. Another more recent avian A(H7N9) virus in poultry and other avian species in China, and the associated human infections, is also of concern.
Other novel respiratory viruses that have emerged recently are the SARS virus in China in 2003 and MERS-CoV, first identified in Saudi Arabia in 2012. Both viruses are Coronaviruses with origins in wild animal species (especially bats). Fortunately SARS was brought under control largely by vigorous implementation of hospital infection control measures. However MERS-CoV continues to cause severe human infections, largely in the Arabian Peninsula, with exposure to camels being a significant risk factor.
Although New Zealand is an island nation, it is very much part of the global community, especially with an economic focus on tourism drawing travellers from all parts of the world. For this reason we need to be aware of infectious diseases and what we can do to work towards their control and ensure that the treatments we have remain effective.
About
Lance Jennings is the Clinical Virologist for the Canterbury District Health Board. He is also a Clinical Associate Professor in the Pathology Department, University of Otago, Christchurch. He has an interest in the diagnosis of clinically relevant human viral infections and the epidemiology, treatment and control of influenza and other respiratory virus infections.
What is InfectedNZ?
Hey, Aotearoa. It’s time we had a chat about infectious diseases and what we’re going to do about the looming antimicrobial armageddon. That’s why we’ve asked leading health, social and economic researchers, and people with personal stories, to help us get real about our vulnerability and discuss solutions. Follow their blogs right here at tepunhahamatatini.ac.nz and watch the conversation spread across social media with #infectedNZ.
Backing it all up, wherever possible, is data from the good folk at Figure.NZ. Their super duper charts are based on data sourced from public repositories, government departments, academics and corporations. Check out their #infectedNZ data board and sign-up to create your very own data board on any topic that floats your boat.
A letter to the Ministry of Health
Below is a letter from Deputy Director Dr Siouxsie Wiles to the Ministry of Health requesting the Ministry’s assistance in using infectious disease data from the Public Health Surveillance website coordinated by the The Institute of Environmental Science and Research Ltd (ESR). The Ministry of Health has responded saying they are looking into the matter.
Dear [Ministry of Health officials]
My name is Siouxsie Wiles and I’m a microbiologist and Senior Lecturer at the University of Auckland. This year I am involved in organising an online campaign called InfectedNZ, to start a national data-driven conversation about infectious diseases. The campaign will run during World Antibiotic Awareness week (November 14-20). The idea is to start thinking about infectious diseases from different perspectives, and to bring together diverse people to look at the available data and write about the situation. The campaign is being run by Te Pūnaha Matatini, a Centre of Research Excellence, and Figure.NZ, a non-profit devoted to getting people to use data about New Zealand. We want to show Aotearoa New Zealand that infectious diseases are a current and future issue with health, social, economic, and environmental impacts.
The importance of our collaboration with Figure.NZ is that they pull together New Zealand’s public sector, private sector and academic data in one place and make it easy for people to use in simple graphical form for free through their website. All data is presented in charts that are designed to be compared easily with each other and constructed with as little bias as possible. Figure.NZ only use data that has a creative commons licence that allows them to publish the data on their site – this is usually the CC BY 3.0 license: https://creativecommons.org/licenses/by/3.0/nz/.
When searching for relevant data on infectious diseases in NZ, I found lots of data presented online on the ESR website (https://surv.esr.cri.nz/), produced under contract with the Ministry of Health. I was particularly interested in obtaining the data to help provide easy to understand charts for experts to use during our campaign. Unfortunately, the current licence allows personal use of the data but not for us to publish on Figure.NZ to help make it easier for people to find and visualise. Instead, there is a disclaimer that instructs users to contact ESR for other uses, such as that envisioned by this campaign (http://www.esr.cri.nz/footer/disclaimer-and-copyright/). We have tried this but the answer was no.
I would like to know what the Ministry of Health’s position is on improving access to publicly available data, like that on the ESR website, for uses like our campaign. We have no interest in manipulating this data other than to standardise the spreadsheets and to present simple visualisations from them. We think that providing usable, clear, digestible and unbiased information will help New Zealanders make better decisions, and will lead to better outcomes for all of us. I am disappointed that important public data like that published online by ESR is essentially out of bounds.
I look forward to your response.
Siouxsie